有机化学品非动物替代风险评估组学研究进展
Advances in Omics Research for Risk Assessment of Non-animal Alternatives to Organic Chemicals
-
摘要: 暴露评估是有机化学品风险评估的关键环节。准确评估有机化学品对动物或人体的危害程度能够有效降低其潜在风险。化学品风险评估的传统方法主要基于动物实验,然而相对于复杂的体内实验,体外细胞毒性实验具有经济、快速、易于量化和可重复的优势。基于人类细胞或细胞系的高通量检测和筛选方法,更有利于表征化学品的毒性效应,满足有机化学品管控的需求。随着生命科学步入后基因组学时代,研究的视角更加广泛,借助于基因组、转录组、蛋白质组、代谢组等组学工具,能够从基因表达的时空分布、蛋白质结构和功能的特性、蛋白质翻译后修饰以及代谢物的动态变化视角为化学品暴露风险评估提供多维度信息。本文从组学角度出发,系统地对近年来国内外有机化学品风险评估的研究进展、组学的研究策略以及组学在有机化学品风险评估中的应用进行了综述和展望,提出组学的联合应用将更加系统全面地探究化学品毒性机制,为我国非动物替代有机化学品暴露风险评估提供技术指引和参考。Abstract: Exposure assessment serves as a pivotal component in the risk evaluation of organic chemicals. Precise evaluation of the potential hazards posed by these chemicals to animals or humans is essential for effectively mitigating their associated risks. Traditional approaches to chemical risk assessment have traditionally relied on animal studies. However, in vitro cytotoxicity experiments present distinct advantages, such as economic efficiency, rapid execution, ease of quantification, and reproducibility, when compared to intricate in vivo experiments. Quantitative automated high-throughput assays, particularly those employing human cells or cell lines, prove advantageous in delineating the toxic effects of chemical substances and addressing the regulatory requirements for controlling organic chemicals. As life sciences transition into the post-genomics era, research perspectives have been broadened. Leveraging omics tools like genomics, transcriptomics, proteomics, and metabolomics enables the provision of multi-dimensional insights for chemical exposure risk assessment. These insights encompass spatial and temporal distributions of gene expression, properties of protein structure and function, post-translational modifications of proteins, and dynamic changes in metabolites. This review systematically examines and outlines recent advancements in organic chemical risk assessment at both domestic and international levels. The review underscores the research strategy of omics and explores its application in organic chemical risk assessment, providing a comprehensive perspective. It is suggested that the collaborative application of omics methodologies will facilitate a more systematic and comprehensive exploration of the toxicity mechanisms of chemicals. Furthermore, this review puts forth technical guidelines and references to support the risk assessment of exposure to non-animal substitute organic chemicals in China.
-
Key words:
- organic chemical substances /
- in vitro risk assessment /
- toxicity test /
- omics techniques
-
-
Sun J C, Fang R C, Wang H, et al. A review of environmental metabolism disrupting chemicals and effect biomarkers associating disease risks: Where exposomics meets metabolomics[J]. Environment International, 2022, 158: 106941 Ahari H, Nowruzi B, Anvar A A, et al. The toxicity testing of cyanobacterial toxins in vivo and in vitro by mouse bioassay: A review[J]. Mini Reviews in Medicinal Chemistry, 2022, 22(8): 1131-1151 Min E K, Lee A N, Lee J Y, et al. Advantages of omics technology for evaluating cadmium toxicity in zebrafish[J]. Toxicological Research, 2021, 37(4): 395-403 Sprenger H, Kreuzer K, Alarcan J, et al. Use of transcriptomics in hazard identification and next generation risk assessment: A case study with clothianidin[J]. Food and Chemical Toxicology, 2022, 166: 113212 刘涛, 郭辰, 赵晓红. 毒理学研究中的体外细胞毒性评价[J]. 生命科学, 2014, 26(3): 319-324 Liu T, Guo C, Zhao X H. In vitro cytotoxicity evaluation in toxicology[J]. Chinese Bulletin of Life Sciences, 2014, 26(3): 319-324(in Chinese)
Bercu J P, Morinello E J, Sehner C, et al. Point of departure (PoD) selection for the derivation of acceptable daily exposures (ADEs) for active pharmaceutical ingredients (APIs)[J]. Regulatory Toxicology and Pharmacology, 2016, 79(Suppl 1): S48-S56 Russell W M S, Burch R L. The principles of humane experimental technique[J]. Medical Journal of Australia, 1960, 1(13): 500 Krewski D, Acosta D Jr, Andersen M, et al. Toxicity testing in the 21st Century: A vision and a strategy[J]. Journal of Toxicology and Environmental Health Part B, Critical Reviews, 2010, 13(2-4): 51-138 Kumar P, Nagarajan A, Uchil P D. Analysis of cell viability by the MTT assay[J]. Cold Spring Harbor Protocols, 2018, 2018(6): 29858338 Batchelor R H, Zhou M J. Use of cellular glucose-6-phosphate dehydrogenase for cell quantitation: Applications in cytotoxicity and apoptosis assays[J]. Analytical Biochemistry, 2004, 329(1): 35-42 Karászi E, Jakab K, Homolya L, et al. Calcein assay for multidrug resistance reliably predicts therapy response and survival rate in acute myeloid leukaemia[J]. British Journal of Haematology, 2001, 112(2): 308-314 Sharma N, Arya G, Kumari R M, et al. Evaluation of anticancer activity of silver nanoparticles on the A549 human lung carcinoma cell lines through alamar blue assay[J]. Bio-protocol, 2019, 9(1): e3131 Adan A, Kiraz Y, Baran Y. Cell proliferation and cytotoxicity assays[J]. Current Pharmaceutical Biotechnology, 2016, 17(14): 1213-1221 Guo M J, Lu B, Gan J L, et al. Apoptosis detection: A purpose-dependent approach selection[J]. Cell Cycle, 2021, 20(11): 1033-1040 Parton R G. Twenty years of traffic: A 2020 vision of cellular electron microscopy[J]. Traffic, 2020, 21(1): 156-161 Buranaamnuay K. The MTT assay application to measure the viability of spermatozoa: A variety of the assay protocols[J]. Open Veterinary Journal, 2021, 11(2): 251-269 Liu K J, Lehmann K P, Sar M, et al. Gene expression profiling following in utero exposure to phthalate esters reveals new gene targets in the etiology of testicular dysgenesis[J]. Biology of Reproduction, 2005, 73(1): 180-192 Shakil M S, Rana Z, Hanif M, et al. Key considerations when using the sulforhodamine B assay for screening novel anticancer agents[J]. Anti-Cancer Drugs, 2022, 33(1): 6-10 Al-Nasiry S, Geusens N, Hanssens M, et al. The use of alamar blue assay for quantitative analysis of viability, migration and invasion of choriocarcinoma cells[J]. Human Reproduction, 2007, 22(5): 1304-1309 Alvarez K L F, Poma-Acevedo A, Fernández-Sánchez M, et al. An EdU-based flow cytometry assay to evaluate chicken T lymphocyte proliferation[J]. BMC Veterinary Research, 2020, 16(1): 230 Jennings P. The future of in vitro toxicology[J]. Toxicology in vitro, 2015, 29(6): 1217-1221 Roden D, McLeod H, Relling M, et al. Pharmacogenomics[J]. The Lancet, 2019, 394: 521-532 van Hummelen P, Sasaki J. State-of-the-art genomics approaches in toxicology[J]. Mutation Research, 2010, 705(3): 165-171 Thomas R S, Allen B C, Nong A, et al. A method to integrate benchmark dose estimates with genomic data to assess the functional effects of chemical exposure[J]. Toxicological Sciences: An Official Journal of the Society of Toxicology, 2007, 98(1): 240-248 Pinheiro E A, Fetterman K A, Burridge P W. hiPSCs in cardio-oncology: Deciphering the genomics[J]. Cardiovascular Research, 2019, 115(5): 935-948 Burridge P W, Li Y F, Matsa E, et al. Human induced pluripotent stem cell-derived cardiomyocytes recapitulate the predilection of breast cancer patients to doxorubicin-induced cardiotoxicity[J]. Nature Medicine, 2016, 22(5): 547-556 Hatherell S, Baltazar M T, Reynolds J, et al. Identifying and characterizing stress pathways of concern for consumer safety in next-generation risk assessment[J]. Toxicological Sciences: An Official Journal of the Society of Toxicology, 2020, 176(1): 11-33 House J S, Grimm F A, Klaren W D, et al. Grouping of UVCB substances with dose-response transcriptomics data from human cell-based assays[J]. ALTEX, 2022, 39(3): 388-404 Liang X F, Martyniuk C J, Simmons D B D. Are we forgetting the “proteomics” in multi-omics ecotoxicology?[J]. Comparative Biochemistry and Physiology Part D: Genomics and Proteomics, 2020, 36: 100751 Lee F, Shah I, Soong Y T, et al. Reproducibility and robustness of high-throughput S1500+ transcriptomics on primary rat hepatocytes for chemical-induced hepatotoxicity assessment[J]. Current Research in Toxicology, 2021, 2: 282-295 Harrill J, Shah I, Setzer R W, et al. Considerations for strategic use of high-throughput transcriptomics chemical screening data in regulatory decisions[J]. Current Opinion in Toxicology, 2019, 15: 64-75 Harrill J A, Everett L J, Haggard D E, et al. High-throughput transcriptomics platform for screening environmental chemicals[J]. Toxicological Sciences: An Official Journal of the Society of Toxicology, 2021, 181(1): 68-89 Webster A F, Chepelev N, Gagné R, et al. Impact of genomics platform and statistical filtering on transcriptional benchmark doses (BMD) and multiple approaches for selection of chemical point of departure (PoD)[J]. PLoS One, 2015, 10(8): e0136764 Titz B, Elamin A, Martin F, et al. Proteomics for systems toxicology[J]. Computational and Structural Biotechnology Journal, 2014, 11(18): 73-90 Prescher J A, Bertozzi C R. Chemical technologies for probing glycans[J]. Cell, 2006, 126(5): 851-854 Zhang Z P, Zhang Y, Li Y, et al. Quantitative phosphoproteomics reveal cellular responses from caffeine, coumarin and quercetin in treated HepG2 cells[J]. Toxicology and Applied Pharmacology, 2022, 449: 116110 Rozanova S, Barkovits K, Nikolov M, et al. Quantitative mass spectrometry-based proteomics: An overview[J]. Methods in Molecular Biology, 2021, 2228: 85-116 Zhang Z R, Wu S, Stenoien D L, et al. High-throughput proteomics[J]. Annual Review of Analytical Chemistry, 2014, 7: 427-454 Carregari V C. Phosphopeptide enrichment techniques: A pivotal step for phosphoproteomic studies[J]. Advances in Experimental Medicine and Biology, 2022, 1382: 17-27 Riley N M, Bertozzi C R, Pitteri S J. A pragmatic guide to enrichment strategies for mass spectrometry-based glycoproteomics[J]. Molecular & Cellular Proteomics, 2021, 20: 100029 Madeira C, Costa P M. Proteomics in systems toxicology[J]. Advances in Protein Chemistry and Structural Biology, 2021, 127: 55-91 Geng H R, Tan X T, Zhao M, et al. Proteomic analysis of zearalenone toxicity on mouse thymic epithelial cells[J]. Journal of Applied Toxicology, 2022, 42(4): 660-670 Li D P, Jiang L L, Hong Y J, et al. Multilayered glycoproteomic analysis reveals the hepatotoxic mechanism in perfluorooctane sulfonate (PFOS) exposure mice[J]. Environmental Pollution, 2021, 268(Pt A): 115774 Caruso J A, Stemmer P M, Dombkowski A, et al. A systems toxicology approach identifies Lyn as a key signaling phosphoprotein modulated by mercury in a B lymphocyte cell model[J]. Toxicology and Applied Pharmacology, 2014, 276(1): 47-54 Sampadi B, Pines A, Munk S, et al. Quantitative phosphoproteomics to unravel the cellular response to chemical stressors with different modes of action[J]. Archives of Toxicology, 2020, 94(5): 1655-1671 Li Y, Zhang Z P, Jiang S H, et al. Using transcriptomics, proteomics and phosphoproteomics as new approach methodology (NAM) to define biological responses for chemical safety assessment[J]. Chemosphere, 2023, 313: 137359 Lindon J C, Holmes E, Bollard M E, et al. Metabonomics technologies and their applications in physiological monitoring, drug safety assessment and disease diagnosis[J]. Biomarkers: Biochemical Indicators of Exposure, Response, and Susceptibility to Chemicals, 2004, 9(1): 1-31 Holmes E, Tang H R, Wang Y L, et al. The assessment of plant metabolite profiles by NMR-based methodologies[J]. Planta Medica, 2006, 72(9): 771-785 Nicholson J K, Lindon J C, Holmes E. 'Metabonomics': Understanding the metabolic responses of living systems to pathophysiological stimuli via multivariate statistical analysis of biological NMR spectroscopic data[J]. Xenobiotica; the Fate of Foreign Compounds in Biological Systems, 1999, 29(11): 1181-1189 Bannuscher A, Hellack B, Bahl A, et al. Metabolomics profiling to investigate nanomaterial toxicity in vitro and in vivo[J]. Nanotoxicology, 2020, 14(6): 807-826 Canzler S, Schor J, Busch W, et al. Prospects and challenges of multi-omics data integration in toxicology[J]. Archives of Toxicology, 2020, 94(2): 371-388 Zhou X, Liu Z H. Unlocking plant metabolic diversity: A (pan)-genomic view[J]. Plant Communications, 2022, 3(2): 100300 Low T Y, Mohtar M A, Lee P Y, et al. Widening the bottleneck of phosphoproteomics: Evolving strategies for phosphopeptide enrichment[J]. Mass Spectrometry Reviews, 2021, 40(4): 309-333 Liu Z X, Wang Y B, Xue Y. Phosphoproteomics-based network medicine[J]. The FEBS Journal, 2013, 280(22): 5696-5704 Olesti E, González-Ruiz V, Wilks M F, et al. Approaches in metabolomics for regulatory toxicology applications[J]. The Analyst, 2021, 146(6): 1820-1834 Ciriello G, Miller M L, Aksoy B A, et al. Emerging landscape of oncogenic signatures across human cancers[J]. Nature Genetics, 2013, 45(10): 1127-1133 Holmgren G, Sartipy P, Andersson C X, et al. Expression profiling of human pluripotent stem cell-derived cardiomyocytes exposed to doxorubicin-integration and visualization of multi-omics data[J]. Toxicological Sciences: An Official Journal of the Society of Toxicology, 2018, 163(1): 182-195 Hsu C H, Tomiyasu H, Liao C H, et al. Genome-wide DNA methylation and RNA-seq analyses identify genes and pathways associated with doxorubicin resistance in a canine diffuse large B-cell lymphoma cell line[J]. PLoS One, 2021, 16(5): e0250013 Schlüter U, Meyer J, Ahrens A, et al. Exposure modelling in Europe: How to pave the road for the future as part of the European Exposure Science Strategy 2020-2030[J]. Journal of Exposure Science & Environmental Epidemiology, 2022, 32(4): 499-512 Brescia S, Alexander-White C, Li H Q, et al. Risk assessment in the 21st Century: Where are we heading?[J]. Toxicology Research, 2023, 12(1): 1-11 Paul Friedman K, Gagne M, Loo L H, et al. Utility of in vitro bioactivity as a lower bound estimate of in vivo adverse effect levels and in risk-based prioritization[J]. Toxicological Sciences, 2020, 173(1): 202-225 Simmons S O, Fan C Y, Ramabhadran R. Cellular stress response pathway system as a sentinel ensemble in toxicological screening[J]. Toxicological Sciences, 2009, 111(2): 202-225 Zhang Q, Bhattacharya S, Pi J B, et al. Adaptive posttranslational control in cellular stress response pathways and its relationship to toxicity testing and safety assessment[J]. Toxicological Sciences: An Official Journal of the Society of Toxicology, 2015, 147(2): 302-316 Bergamini G, Bell K, Shimamura S, et al. A selective inhibitor reveals PI3Kγ dependence of TH17 cell differentiation[J]. Nature Chemical Biology, 2012, 8: 576-582 Hendriks G, Derr R S, Misovic B, et al. The extended ToxTracker assay discriminates between induction of DNA damage, oxidative stress, and protein misfolding[J]. Toxicological Sciences: An Official Journal of the Society of Toxicology, 2016, 150(1): 190-203 Baltazar M T, Cable S, Carmichael P L, et al. A next-generation risk assessment case study for coumarin in cosmetic products[J]. Toxicological Sciences: An Official Journal of the Society of Toxicology, 2020, 176(1): 236-252 Martins C, Dreij K, Costa P M. The state-of-the art of environmental toxicogenomics: Challenges and perspectives of “omics” approaches directed to toxicant mixtures[J]. International Journal of Environmental Research and Public Health, 2019, 16(23): 4718 Portugal J, Mansilla S, Piña B. Perspectives on the use of toxicogenomics to assess environmental risk[J]. Frontiers in Bioscience (Landmark Edition), 2022, 27(10): 294 Vaquerizas J M, Kummerfeld S K, Teichmann S A, et al. A census of human transcription factors: Function, expression and evolution[J]. Nature Reviews Genetics, 2009, 10: 252-263 Satoda N, Shoji T, Wu Y L, et al. Value of FOXP3 expression in peripheral blood as rejection marker after miniature swine lung transplantation[J]. The Journal of Heart and Lung Transplantation: The Official Publication of the International Society for Heart Transplantation, 2008, 27(12): 1293-1301 Niu N, Xu S W, Xu Y N, et al. Targeting mechanosensitive transcription factors in atherosclerosis[J]. Trends in Pharmacological Sciences, 2019, 40(4): 253-266 Sauer U G, Deferme L, Gribaldo L, et al. The challenge of the application of omics technologies in chemicals risk assessment: Background and outlook[J]. Regulatory Toxicology and Pharmacology, 2017, 91(Suppl 1): S14-S26 Escher S E, Kamp H, Bennekou S H, et al. Towards grouping concepts based on new approach methodologies in chemical hazard assessment: The read-across approach of the EU-ToxRisk project[J]. Archives of Toxicology, 2019, 93(12): 3643-3667 Salit M, Woodcock J. MAQC and the era of genomic medicine[J]. Nature Biotechnology, 2021, 39(9): 1066-1067 Marx-Stoelting P, Braeuning A, Buhrke T, et al. Application of omics data in regulatory toxicology: Report of an international BfR expert workshop[J]. Archives of Toxicology, 2015, 89(11): 2177-2184 Carusi A, Davies M R, De Grandis G, et al. Harvesting the promise of AOPs: An assessment and recommendations[J]. The Science of the Total Environment, 2018, 628-629: 1542-1556 Tebby C, Gao W, Delp J, et al. A quantitative AOP of mitochondrial toxicity based on data from three cell lines[J]. Toxicology in vitro, 2022, 81: 105345 Ankley G T, Bennett R S, Erickson R J, et al. Adverse outcome pathways: A conceptual framework to support ecotoxicology research and risk assessment[J]. Environmental Toxicology and Chemistry, 2010, 29(3): 730-741 Burden N, Sewell F, Andersen M E, et al. Adverse outcome pathways can drive non-animal approaches for safety assessment[J]. Journal of Applied Toxicology, 2015, 35(9): 971-975 Maxwell G, MacKay C, Cubberley R, et al. Applying the skin sensitisation adverse outcome pathway (AOP) to quantitative risk assessment[J]. Toxicology in vitro: An International Journal Published in Association with BIBRA, 2014, 28(1): 8-12 Spassova M A. Statistical approach to identify threshold and point of departure in dose-response data[J]. Risk Analysis: An Official Publication of the Society for Risk Analysis, 2019, 39(4): 940-956 Spassova M A, Miller D J, Nikolov A S. Kinetic modeling reveals the roles of reactive oxygen species scavenging and DNA repair processes in shaping the dose-response curve of KBrO3-induced DNA damage[J]. Oxidative Medicine and Cellular Longevity, 2015, 2015: 764375 -
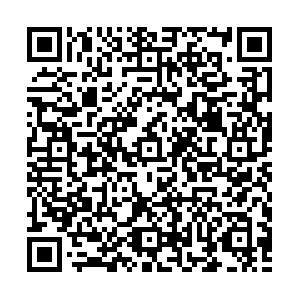
计量
- 文章访问数: 1205
- HTML全文浏览数: 1205
- PDF下载数: 134
- 施引文献: 0