海洋酸化对藻类生态学效应及作用机制的研究进展
Research Progress on Ecological Effects and Mechanism of Ocean Acidification on Algae
-
摘要: 目前全球海洋酸化(ocean acidification, OA)问题正在以前所未有的速度快速恶化,使海洋生物以及海洋生态环境面临着巨大的威胁。藻类作为海洋中最主要的初级生产者,贡献了约95%的初级生产力,是物质循环和能量流动的重要环节。海洋酸化能够改变藻类的初级生产力,从而影响海洋食物网中物质和能量从初级到次级生产者及更高营养级的传递,引发食物链效应,进而对整个海洋生态系统带来不可逆转的影响,因此评估海洋酸化对藻类的影响具有重要的生态学研究意义。本文总结了近年来海洋酸化对藻类的光合作用、碳固定、生长、钙化、繁殖等生理生化过程以及对代谢组分和显微结构的影响,归纳了海洋酸化对藻类的分子调控机制,同时围绕海洋酸化与环境因子以及海洋污染物对藻类的复合胁迫展开综述,并基于当前海洋酸化对藻类影响研究中存在的不足做出展望,以期为人们解决海洋酸化问题提供思路和方法。Abstract: Presently, ocean acidification (OA) is escalating at an unprecedented pace, posing a considerable threat to the chemical equilibrium of seawater. This acceleration has the potential to disrupt the marine ecological environment significantly, affecting various organisms. Among these, algae, the primary producers in the ocean, contribute 95% of productivity and play a vital role in material and energy cycling. The increasing acidity in the ocean could alter algae productivity, subsequently impacting material and energy transfer through the marine food web. Such changes may initiate a cascade effect in the food chain, leading to irreversible damage to the entire marine ecosystem. Additionally, algae's heightened sensitivity to environmental changes makes them valuable as “indicator organisms” for monitoring water quality. Therefore, assessing the ecological impact of ocean acidification on algae is crucial for ecological research. This review aims to outline the effects of ocean acidification on the physiological and biochemical processes of algae over recent years. This includes aspects such as photosynthesis, inorganic carbon fixation, growth, calcification, reproduction, as well as metabolic components and microstructure. The review also explores how ocean acidification influences the molecular regulation mechanisms of algae, considering its combined effects with environmental factors and marine pollutants. Furthermore, the article analyzes the limitations of current algae research on ocean acidification and proposes solutions and strategies to address this pressing issue.
-
Key words:
- ocean acidification /
- algae /
- ecological effects /
- mechanism of action
-
-
International Energy Agency. Global Energy Review 2021[R]. Paris: International Energy Agency, 2021 Landschützer P, Gruber N, Bakker D C E, et al. Recent variability of the global ocean carbon sink[J]. Global Biogeochemical Cycles, 2014, 28(9): 927-949 Orr J C, Fabry V J, Aumont O, et al. Anthropogenic ocean acidification over the twenty-first century and its impact on calcifying organisms[J]. Nature, 2005, 437(7059): 681-686 Xu H T, Li L A, Wang Y J, et al. Differential physiological response of marine and freshwater microalgae to polystyrene microplastics[J]. Journal of Hazardous Materials, 2023, 448: 130814 Li S N, Li X, Ho S H. How to enhance carbon capture by evolution of microalgal photosynthesis?[J]. Separation and Purification Technology, 2022, 291: 120951 Trimborn S, Thoms S, Brenneis T, et al. Two Southern Ocean diatoms are more sensitive to ocean acidification and changes in irradiance than the prymnesiophyte Phaeocystis antarctica[J]. Physiologia Plantarum, 2017, 160(2): 155-170 邹定辉, 陈雄文. 高浓度CO2对条浒苔(Enteromorpha clathrata)生长和一些生理生化特征的影响[J]. 海洋通报, 2002, 21(5): 38-45 Zou D H, Chen X W. Effects of elevated CO2 concentration on growth and some physiological and biochemical traits in Enteromorpha clathrata (Chlorophyta)[J]. Marine Science Bulleten, 2002, 21(5): 38-45(in Chinese)
Hu S X, Zhou B, Wang Y, et al. Effect of CO2-induced seawater acidification on growth, photosynthesis and inorganic carbon acquisition of the harmful bloom-forming marine microalga, Karenia mikimotoi[J]. PLoS One, 2017, 12(8): e0183289 Li H X, Xu T P, Ma J, et al. Physiological responses of Skeletonema costatum to the interactions of seawater acidification and the combination of photoperiod and temperature[J]. Biogeosciences, 2021, 18(4): 1439-1449 陈斌斌. 海洋酸化背景下经济海藻龙须菜与坛紫菜的生物学特性[D]. 广州: 华南理工大学, 2015: 47-54 Chen B B. Biological adaptation of Gracilaria lemaneiformis and Pyropia haitanensis affected by ocean acidification[D]. Guangzhou: South China University of Technology, 2015: 47 -54(in Chinese)
Raven J A. Limits on growth rates[J]. Nature, 1993, 361: 209-210 Heureux A M C, Young J N, Whitney S M, et al. The role of Rubisco kinetics and pyrenoid morphology in shaping the CCM of haptophyte microalgae[J]. Journal of Experimental Botany, 2017, 68(14): 3959-3969 Raven J A, Beardall J, Sánchez-Baracaldo P. The possible evolution and future of CO2-concentrating mechanisms[J]. Journal of Experimental Botany, 2017, 68(14): 3701-3716 Shi D L, Hong H Z, Su X, et al. The physiological response of marine diatoms to ocean acidification: Differential roles of seawater pCO2 and pH[J]. Journal of Phycology, 2019, 55(3): 521-533 Chen X W, Gao K S. Effect of CO2 concentrations on the activity of photosynthetic CO2 fixation and extracelluar carbonic anhydrase in the marine diatom Skeletonema costatum[J]. Chinese Science Bulletin, 2003, 48(23): 2616-2620 Zoccola D, Innocenti A, Bertucci A, et al. Coral carbonic anhydrases: Regulation by ocean acidification[J]. Marine Drugs, 2016, 14(6): 109 Qiu B S, Liu J Y. Utilization of inorganic carbon in the edible cyanobacterium Ge-Xian-Mi (Nostoc) and its role in alleviating photo-inhibition[J]. Plant, Cell & Environment, 2004, 27(12): 1447-1458 Paine E R, Britton D, Schmid M, et al. No effect of ocean acidification on growth, photosynthesis, or dissolved organic carbon release by three temperate seaweeds with different dissolved inorganic carbon uptake strategies[J]. ICES Journal of Marine Science, 2023, 80(2): 272-281 Hennon G M, Quay P, Morales R L, et al. Acclimation conditions modify physiological response of the diatom Thalassiosira pseudonana to elevated CO2 concentrations in a nitrate-limited chemostat[J]. Journal of Phycology, 2014, 50(2): 243-253 郝爽. 海洋酸化对微小亚历山大藻产毒的影响和调控机制初探[D]. 济南: 山东大学, 2021: 27-41 Hao S. Study on the effect of ocean acidification on the toxin production of Alexandrium parvum and its regulation mechanism[D]. Jinan: Shandong University, 2021: 27 -41(in Chinese)
范佳乐, 李富田, 徐军田. 海水酸化和碱化对斑点海链藻光合生理特性的影响[J]. 海洋学报, 2020, 42(12): 62-71 Fan J L, Li F T, Xu J T. Effect of seawater acidification and alkalization on photosynthetic physiology of Thalassiosira punctigera[J]. Haiyang Xuebao, 2020, 42(12): 62-71(in Chinese)
郑伟, 钟志海, 杨梓, 等. 大气CO2增加对不同生长光强下龙须菜光合生理特性的影响[J]. 生态学报, 2014, 34(24): 7293-7299 Zheng W, Zhong Z H, Yang Z, et al. Effects of elevated CO2 concentration on the photosynthetic physiological characteristics of Gracilaria lemaneiformis grown under different light levels[J]. Acta Ecologica Sinica, 2014, 34(24): 7293-7299(in Chinese)
常思伟. 区分海洋酸化过程中二氧化碳分压上升和pH下降对束毛藻的影响及机理初探[D]. 厦门: 厦门大学, 2017: 41-42 Chang S W. Dissecting the effect of pCO2 and pH on Trichodesmium IMS 101 and its mechanisms[D]. Xiamen: Xiamen University, 2017: 41-42(in Chinese)
Kottmeier D M, Rokitta S D, Rost B. H+-driven increase in CO2 uptake and decrease in HCO3- uptake explain coccolithophores' acclimation responses to ocean acidification[J]. Limnology and Oceanography, 2016, 61(6): 2045-2057 Feng Y Y, Roleda M Y, Armstrong E, et al. Effects of multiple drivers of ocean global change on the physiology and functional gene expression of the coccolithophore Emiliania huxleyi[J]. Global Change Biology, 2020, 26(10): 5630-5645 Riebesell U, Zondervan I, Rost B, et al. Reduced calcification of marine plankton in response to increased atmospheric CO2[J]. Nature, 2000, 407(6802): 364-367 韦章良, 莫嘉豪, 胡群菊, 等. 不同光照强度下仙掌藻(Halimeda opuntia)对海洋酸化的生理响应[J]. 海洋通报, 2019, 38(5): 574-584 Wei Z L, Mo J H, Hu Q J, et al. The physiological performance of the calcifying green macroalga Halimeda opuntia in response to ocean acidification with irradiance variability[J]. Marine Science Bulletin, 2019, 38(5): 574-584(in Chinese)
Diner R E, Benner I, Passow U, et al. Negative effects of ocean acidification on calcification vary within the coccolithophore genus Calcidiscus[J]. Marine Biology, 2015, 162(6): 1287-1305 Iglesias-Rodriguez M D, Halloran P R, Rickaby R E, et al. Phytoplankton calcification in a high-CO2 world[J]. Science, 2008, 320(5874): 336-340 Shi D L, Xu Y K, Morel F M M. Effects of the pH/pCO2 control method on medium chemistry and phytoplankton growth[J]. Biogeosciences, 2009, 6(7): 1199-1207 Benner I, Diner R E, Lefebvre S C, et al. Emiliania huxleyi increases calcification but not expression of calcification-related genes in long-term exposure to elevated temperature and pCO2[J]. Philosophical Transactions of the Royal Society of London Series B, Biological Sciences, 2013, 368(1627): 20130049 Lohbeck K T, Riebesell U, Reusch T B H. Gene expression changes in the coccolithophore Emiliania huxleyi after 500 generations of selection to ocean acidification[J]. Proceedings Biological Sciences, 2014, 281(1786): 20140003 Fiorini S, Middelburg J J, Gattuso J P. Effects of elevated CO2 partial pressure and temperature on the coccolithophore Syracosphaera pulchra[J]. Aquatic Microbial Ecology, 2011, 64(3): 221-232 González C P, Edding M, Tala F, et al. Exposure time modulates the effects of climate change-related stressors on fertile sporophytes and early-life stage performance of a habitat-forming kelp species[J]. Environmental Pollution, 2021, 286: 117224 Roleda M Y, Morris J N, McGraw C M, et al. Ocean acidification and seaweed reproduction: Increased CO2 ameliorates the negative effect of lowered pH on meiospore germination in the giant kelp Macrocystis pyrifera (Laminariales, Phaeophyceae)[J]. Global Change Biology, 2012, 18(3): 854-864 Gaitán-Espitia J D, Hancock J R, Padilla-Gamiño J L, et al. Interactive effects of elevated temperature and pCO2 on early-life-history stages of the giant kelp Macrocystis pyrifera[J]. Journal of Experimental Marine Biology and Ecology, 2014, 457: 51-58 Xu D, Wang D S, Li B, et al. Effects of CO2 and seawater acidification on the early stages of Saccharina japonica development[J]. Environmental Science & Technology, 2015, 49(6): 3548-3556 Ragazzola F, Foster L C, Form A, et al. Ocean acidification weakens the structural integrity of coralline algae[J]. Global Change Biology, 2012, 18(9): 2804-2812 González C P, Edding M, Torres R, et al. Increased temperature but not pCO2 levels affect early developmental and reproductive traits of the economically important habitat-forming kelp Lessonia trabeculata[J]. Marine Pollution Bulletin, 2018, 135: 694-703 Redmond S. Effects of increasing temperature and ocean acidification on the microstages of two populations of Saccharina latissima in the northwest Atlantic[D]. New Britain: University of Connecticut, 2013: 1-51 Bermúdez R, Feng Y Y, Roleda M Y, et al. Long-term conditioning to elevated pCO2 and warming influences the fatty and amino acid composition of the diatom Cylindrotheca fusiformis[J]. PLoS One, 2015, 10(5): e0123945 Moghimifam R, Niknam V, Ebrahimzadeh H, et al. The influence of different CO2 concentrations on the biochemical and molecular response of two isolates of Dunaliella sp. (ABRIINW-CH2 and ABRIINW-SH33)[J]. Journal of Applied Phycology, 2020, 32(1): 175-187 Tang D H, Han W, Li P L, et al. CO2 biofixation and fatty acid composition of Scenedesmus obliquus and Chlorella pyrenoidosa in response to different CO2 levels[J]. Bioresource Technology, 2011, 102(3): 3071-3076 Wang X W, Liang J R, Luo C S, et al. Biomass, total lipid production, and fatty acid composition of the marine diatom Chaetoceros muelleri in response to different CO2 levels[J]. Bioresource Technology, 2014, 161: 124-130 Liang C W, Zhang Y F, Wang L, et al. Features of metabolic regulation revealed by transcriptomic adaptions driven by long-term elevated pCO2 in Chaetoceros muelleri[J]. Phycological Research, 2020, 68(3): 236-248 Adjout R, Mouget J L, Pruvost J, et al. Effects of temperature, irradiance, and pH on the growth and biochemical composition of Haslea ostrearia batch-cultured in an airlift plan-photobioreactor[J]. Applied Microbiology and Biotechnology, 2022, 106(13-16): 5233-5247 Qiu R H, Gao S, Lopez P A, et al. Effects of pH on cell growth, lipid production and CO2 addition of microalgae Chlorella sorokiniana[J]. Algal Research, 2017, 28: 192-199 Ogbonda K H, Aminigo R E, Abu G O. Influence of temperature and pH on biomass production and protein biosynthesis in a putative Spirulina sp.[J]. Bioresource Technology, 2007, 98(11): 2207-2211 Khalil Z I, Asker M M, El-Sayed S, et al. Effect of pH on growth and biochemical responses of Dunaliella bardawil and Chlorella ellipsoidea [J]. World Journal of Microbiology & Biotechnology, 2010, 26(7): 1225-1231 杨英. CO2浓度升高对微藻类生长和光合作用影响的研究[D]. 汕头: 汕头大学, 2000: 41-45 Yang Y. Effects of elevated CO2 concentration on growth and photosynthesis of microalgae[D]. Shantou: Shantou University, 2000 : 41-45(in Chinese)
夏建荣, 高坤山. 不同CO2浓度下培养的蛋白核小球藻细胞结构的变化[J]. 武汉植物学研究, 2002, 20(5): 403-404 Xia J R, Gao K S. Change of cell structure of Chlorella pyrenoidosa grown under different CO2 concentration[J]. Journal of Wuhan Botanical Research, 2002, 20(5): 403-404(in Chinese)
Miyachi S, Tsuzuki M, Maruyama I, et al. Effects of CO2 concentration during growth on the intracellular structure of Chlorella and Scenedesmus (Chlorophyta)[J]. Journal of Phycology, 1986, 22(3): 313-319 Stefanov M A, Rashkov G D, Yotsova E K, et al. Impact of salinity on the energy transfer between pigment-protein complexes in photosynthetic apparatus, functions of the oxygen-evolving complex and photochemical activities of photosystem Ⅱ and photosystem Ⅰ in two Paulownia lines[J]. International Journal of Molecular Sciences, 2023, 24(4): 3108 王璐. 水体酸化对几种微藻显微结构和营养成分的影响[D]. 青岛: 青岛科技大学, 2020: 21-22 Wang L. Effects of water acidification on microstructure and nutritional components of several microalgae[D]. Qingdao: Qingdao University of Science & Technology, 2020: 21 -22(in Chinese)
Kumar A, Castellano I, Patti F P, et al. Molecular response of Sargassum vulgare to acidification at volcanic CO2 vents: Insights from de novo transcriptomic analysis[J]. Molecular Ecology, 2017, 26(8): 2276-2290 Ye X, Chen J N, Hu C Y, et al. Promotion of the rapid growth in Haematococcus pluvialis under 0.16% CO2 condition revealed by transcriptome and metabolomic analysis[J]. Journal of Plant Growth Regulation, 2020, 39(3): 1177-1190 Tan Y H, Poong S W, Yang C H, et al. Transcriptomic analysis reveals distinct mechanisms of adaptation of a polar picophytoplankter under ocean acidification conditions[J]. Marine Environmental Research, 2022, 182: 105782 Liang C W, Zhang Y F, Gu Z P, et al. Elevated pCO2 induced physiological, molecular and metabolic changes in Nannochloropsis oceanica and its effects on trophic transfer[J]. Frontiers in Marine Science, 2022, 9: 863262 Huang B, Qu G P, He Y L, et al. Study on high-CO2 tolerant Dunaliella salina and its mechanism via transcriptomic analysis[J]. Frontiers in Bioengineering and Biotechnology, 2022, 10: 1086357 Sasaki T, Pronina N, Maeshima M, et al. Development of vacuoles and vacuolar H+-ATPase activity under extremely high CO2 conditions in Chlorococcum littorale cells[J]. Plant Biology, 1999, 1(1): 68-75 Dietz K J, Tavakoli N, Kluge C, et al. Significance of the V-type ATPase for the adaptation to stressful growth conditions and its regulation on the molecular and biochemical level[J]. Journal of Experimental Botany, 2001, 52(363): 1969-1980 Rokitta S D, John U, Rost B. Ocean acidification affects redox-balance and ion-homeostasis in the life-cycle stages of Emiliania huxleyi[J]. PLoS One, 2012, 7(12): e52212 Thangaraj S, Sun J. Ocean warming and acidification affect the transitional C∶N∶P ratio and macromolecular accumulation in the harmful raphidophyte Heterosigma akashiwo[J]. Communications Biology, 2023, 6(1): 151 Liu L, Zou D H, Jiang H, et al. Effects of increased CO2 and temperature on the growth and photosynthesis in the marine macroalga Gracilaria lemaneiformis from the coastal waters of South China[J]. Journal of Applied Phycology, 2018, 30(2): 1271-1280 Nagao R, Ueno Y, Akimoto S, et al. Effects of CO2 and temperature on photosynthetic performance in the diatom Chaetoceros gracilis[J]. Photosynthesis Research, 2020, 146(1-3): 189-195 Thangaraj S, Sun J. The biotechnological potential of the marine diatom Skeletonema dohrnii to the elevated temperature and pCO2 concentration[J]. Marine Drugs, 2020, 18(5): 259 Fu F X, Warner M E, Zhang Y H, et al. Effects of increased temperature and CO2 on photosynthesis, growth, and elemental ratios in marine Synechococcus and Prochlorococcus (Cyanobacteria)[J]. Journal of Phycology, 2007, 43(3): 485-496 黄征征, 黄旭雄, 严佳琦, 等. 生长阶段及温度对微藻细胞总ATP酶活性的影响[J]. 海洋渔业, 2011, 33(2): 181-186 Huang Z Z, Huang X X, Yan J Q, et al. Effects of growth phase and temperature on the total ATPase activity of microalgae[J]. Marine Fisheries, 2011, 33(2): 181-186(in Chinese)
Passow U, Laws E A. Ocean acidification as one of multiple stressors: Growth response of Thalassiosira weissflogii (diatom) under temperature and light stress[J]. Marine Ecology Progress Series, 2015, 541: 75-90 Rastogi R P, Singh S P, Häder D P, et al. Ultraviolet-B-induced DNA damage and photorepair in the Cyanobacterium Anabaena variabilis PCC 7937[J]. Environmental and Experimental Botany, 2011, 74: 280-288 Gao K S, Ruan Z X, Villafañe V E, et al. Ocean acidification exacerbates the effect of UV radiation on the calcifying phytoplankter Emiliania huxleyi[J]. Limnology and Oceanography, 2009, 54(6): 1855-1862 Li F T, Li H X, Xu T P, et al. Seawater acidification exacerbates the negative effects of UVR on the growth of the bloom-forming diatom Skeletonema costatum [J]. Frontiers in Marine Science, 2022, 9: 905255 Chen S W, Gao K S. Solar ultraviolet radiation and CO2-induced ocean acidification interacts to influence the photosynthetic performance of the red tide alga Phaeocystis globosa (Prymnesiophyceae)[J]. Hydrobiologia, 2011, 675(1): 105-117 García-Gómez C, Mata M T, Van Breusegem F, et al. Low-steady-state metabolism induced by elevated CO2 increases resilience to UV radiation in the unicellular green-algae Dunaliella tertiolecta[J]. Environmental and Experimental Botany, 2016, 132: 163-174 Ma J, Wang W, Liu X Y, et al. Zinc toxicity alters the photosynthetic response of red alga Pyropia yezoensis to ocean acidification[J]. Environmental Science and Pollution Research International, 2020, 27(3): 3202-3212 de Orte M R, Sarmiento A M, Basallote M D, et al. Effects on the mobility of metals from acidification caused by possible CO2 leakage from sub-seabed geological formations[J]. The Science of the Total Environment, 2014, 470-471: 356-363 Dong F, Wang P, Qian W, et al. Mitigation effects of CO2-driven ocean acidification on Cd toxicity to the marine diatom Skeletonema costatum[J]. Environmental Pollution, 2020, 259: 113850 Xu D, Huang S J, Fan X, et al. Elevated CO2 reduces copper accumulation and toxicity in the diatom Thalassiosira pseudonana[J]. Frontiers in Microbiology, 2022, 13: 1113388 Payán M C, Verbinnen B, Galan B, et al. Potential influence of CO2 release from a carbon capture storage site on release of trace metals from marine sediment[J]. Environmental Pollution, 2012, 162: 29-39 Millero F, Woosley R, DiTrolio B, et al. Effect of ocean acidification on the speciation of metals in seawater[J]. Oceanography, 2009, 22(4): 72-85 Xu T P, Cao J Y, Qian R, et al. Ocean acidification exacerbates copper toxicity in both juvenile and adult stages of the green tide alga Ulva linza[J]. Marine Environmental Research, 2021, 170: 105447 Gao G, Liu Y M, Li X S, et al. Expected CO2-induced ocean acidification modulates copper toxicity in the green tide alga Ulva prolifera[J]. Environmental and Experimental Botany, 2017, 135: 63-72 陈灿, 杨黎彬, 周雪飞. 纳米材料对水环境中微藻毒性的效应及机理研究进展[J]. 净水技术, 2022, 41(5): 5-13 Chen C, Yang L B, Zhou X F. Research progress of effect and mechanism of nanomaterials on microalgae toxicity in water environment[J]. Water Purification Technology, 2022, 41(5): 5-13(in Chinese)
Sjollema S B, Redondo-Hasselerharm P, Leslie H A, et al. Do plastic particles affect microalgal photosynthesis and growth?[J]. Aquatic Toxicology, 2016, 170: 259-261 Fotopoulou K N, Karapanagioti H K. Surface properties of beached plastic pellets[J]. Marine Environmental Research, 2012, 81: 70-77 Ren Y D, Jia Z H, Liu Y J, et al. Elevated pCO2 alleviates the toxic effects of polystyrene nanoparticles on the marine microalga Nannochloropsis oceanica[J]. The Science of the Total Environment, 2023, 895: 164985 Lagarde F, Olivier O, Zanella M, et al. Microplastic interactions with freshwater microalgae: Hetero-aggregation and changes in plastic density appear strongly dependent on polymer type[J]. Environmental Pollution, 2016, 215: 331-339 -
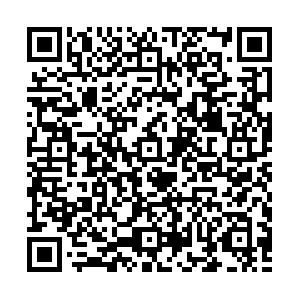
计量
- 文章访问数: 3131
- HTML全文浏览数: 3131
- PDF下载数: 263
- 施引文献: 0