合成纳米材料对神经系统的影响
Effects of Engineered Nanomaterials on Nervous System
-
摘要: 合成纳米材料具有独特的物理化学特性,在能源、电子、食品、农业、环境科学、化妆品和医药等领域都得到广泛应用,与此同时其安全性也受到了广泛关注。体内和体外模型研究已报道合成纳米材料能够穿过或绕过血脑屏障,进入中枢神经系统,造成神经损伤。本文总结了合成纳米材料产生毒性的影响因素,进入中枢神经系统的途径以及诱导神经毒性的分子机制,包括氧化应激、DNA损伤、炎症、自噬和凋亡的诱导以及DNA甲基化等。通过系统地综述合成纳米材料神经毒性研究进展,并对该研究方向进行展望,为进一步深入研究合成纳米材料神经毒性提供参考。Abstract: Due to their unique physicochemical properties, engineered nanomaterials have been widely used in various fields, including energy, electronics, food and agriculture, environmental science, cosmetics, and medicine, etc. In the meantime, much attention has been paid on the safety of engineered nanomaterials. Recently, in vivo and in vitro studies have proven that engineered nanomaterials can cross or bypass the blood-brain barrier and then access the central nervous system, causing neurotoxicity. In this paper, the pathway of engineered nanomaterials crossing the central nervous system (CNS), the neurotoxicity and potential molecular mechanisms of engineered nanomaterials, as well as the effect of engineered nanomaterials physicochemical properties on the toxicity have been summarized. Herein, the potential mechanisms includes oxidative stress, DNA damage, inflammation, autophagy, apoptosis and DNA methylation. The remarkable research progress and perspectives are highlighted in this review, boosting further in-depth neurotoxicity research of engineered nanomaterials.
-
-
Teleanu D M, Chircov C, Grumezescu A M, et al. Neurotoxicity of nanomaterials:An up-to-date overview[J]. Nanomaterials, 2019, 9(1):E96 Keller A A, Lazareva A. Predicted releases of engineered nanomaterials:From global to regional to local[J]. Environmental Science & Technology Letters, 2014, 1(1):65-70 Weir A, Westerhoff P, Fabricius L, et al. Titanium dioxide nanoparticles in food and personal care products[J]. Environmental Science & Technology, 2012, 46(4):2242-2250 Gendelman H E, Mosley R L, Boska M D, et al. The promise of nanoneuromedicine[J]. Nanomedicine, 2014, 9(2):171-176 Siddiqi K S, Husen A, Sohrab S S, et al. Recent status of nanomaterial fabrication and their potential applications in neurological disease management[J]. Nanoscale Research Letters, 2018, 13(1):231 Chang X R, Li J Y, Niu S Y, et al. Neurotoxicity of metal-containing nanoparticles and implications in glial cells[J]. Journal of Applied Toxicology, 2021, 41(1):65-81 徐莺莺, 林晓影, 陈春英. 影响纳米材料毒性的关键因素[J]. 科学通报, 2013, 58(24):2466-2478 Xu Y Y, Lin X Y, Chen C Y. Key factors influencing the toxicity of nanomaterials[J]. Chinese Science Bulletin, 2013, 58(24):2466-2478(in Chinese)
Barhoum A, García-Betancourt M L, Jeevanandam J, et al. Review on natural, incidental, bioinspired, and engineered nanomaterials:History, definitions, classifications, synthesis, properties, market, toxicities, risks, and regulations[J]. Nanomaterials, 2022, 12(2):177 Nel A, Xia T, Mädler L, et al. Toxic potential of materials at the nanolevel[J]. Science, 2006, 311(5761):622-627 Zhao F, Zhao Y, Liu Y, et al. Cellular uptake, intracellular trafficking, and cytotoxicity of nanomaterials[J]. Small, 2011, 7(10):1322-1337 Park E J, Bae E, Yi J, et al. Repeated-dose toxicity and inflammatory responses in mice by oral administration of silver nanoparticles[J]. Environmental Toxicology and Pharmacology, 2010, 30(2):162-168 Champion J A, Mitragotri S. Role of target geometry in phagocytosis[J]. Proceedings of the National Academy of Sciences of the United States of America, 2006, 103(13):4930-4934 Chithrani B D, Ghazani A A, Chan W C W. Determining the size and shape dependence of gold nanoparticle uptake into mammalian cells[J]. Nano Letters, 2006, 6(4):662-668 Senthilkumar N, Sharma P K, Sood N, et al. Designing magnetic nanoparticles for in vivo applications and understanding their fate inside human body[J]. Coordination Chemistry Reviews, 2021, 445:214082 Qiu Y, Liu Y, Wang L M, et al. Surface chemistry and aspect ratio mediated cellular uptake of Au nanorods[J]. Biomaterials, 2010, 31(30):7606-7619 Li Y Y, Zhou Y L, Wang H Y, et al. Chirality of glutathione surface coating affects the cytotoxicity of quantum dots[J]. Angewandte Chemie, 2011, 50(26):5860-5864 Zhai W Y, He C L, Wu L, et al. Degradation of hollow mesoporous silica nanoparticles in human umbilical vein endothelial cells[J]. Journal of Biomedical Materials Research Part B, Applied Biomaterials, 2012, 100(5):1397-1403 Chiou B, Connor J R. Emerging and dynamic biomedical uses of ferritin[J]. Pharmaceuticals, 2018, 11(4):124 Qu Y, Li W, Zhou Y L, et al. Full assessment of fate and physiological behavior of quantum dots utilizing Caenorhabditis elegans as a model organism[J]. Nano Letters, 2011, 11(8):3174-3183 Gliga A R, Skoglund S, Wallinder I O, et al. Size-dependent cytotoxicity of silver nanoparticles in human lung cells:The role of cellular uptake, agglomeration and Ag release[J]. Particle and Fibre Toxicology, 2014, 11:11 Ge D, Du Q Q, Ran B Q, et al. The neurotoxicity induced by engineered nanomaterials[J]. International Journal of Nanomedicine, 2019, 14:4167-4186 Ballabh P, Braun A, Nedergaard M. The blood-brain barrier:An overview:Structure, regulation, and clinical implications[J]. Neurobiology of Disease, 2004, 16(1):1-13 Karmakar A, Zhang Q L, Zhang Y B. Neurotoxicity of nanoscale materials[J]. Journal of Food and Drug Analysis, 2014, 22(1):147-160 Lovisolo D, Dionisi M, Ruffinatti F A, et al. Nanoparticles and potential neurotoxicity:Focus on molecular mechanisms[J]. AIMS Molecular Science, 2018, 5(1):1-13 Yamashita K, Yoshioka Y, Higashisaka K, et al. Silica and titanium dioxide nanoparticles cause pregnancy complications in mice[J]. Nature Nanotechnology, 2011, 6(5):321-328 Korzeniowska B, Fonseca M P, Gorshkov V, et al. The cytotoxicity of metal nanoparticles depends on their synergistic interactions[J]. Particle & Particle Systems Characterization, 2020, 37(8):2000135 Hu Q L, Guo F L, Zhao F H, et al. Effects of titanium dioxide nanoparticles exposure on Parkinsonism in zebrafish larvae and PC12[J]. Chemosphere, 2017, 173:373-379 Lv M, Zhang Y J, Liang L, et al. Effect of graphene oxide on undifferentiated and retinoic acid-differentiated SH-SY5Y cells line[J]. Nanoscale, 2012, 4(13):3861-3866 Guo Z L, Zhang P, Chetwynd A J, et al. Elucidating the mechanism of the surface functionalization dependent neurotoxicity of graphene family nanomaterials[J]. Nanoscale, 2020, 12(36):18600-18605 Wei L M, Wang J F, Chen A J, et al. Involvement of PINK1/parkin-mediated mitophagy in ZnO nanoparticle-induced toxicity in BV-2 cells[J]. International Journal of Nanomedicine, 2017, 12:1891-1903 Sharma A K, Singh V, Gera R, et al. Zinc oxide nanoparticle induces microglial death by NADPH-oxidase-independent reactive oxygen species as well as energy depletion[J]. Molecular Neurobiology, 2017, 54(8):6273-6286 Li P, Xu T T, Wu S Y, et al. Chronic exposure to graphene-based nanomaterials induces behavioral deficits and neural damage in Caenorhabditis elegans[J]. Journal of Applied Toxicology, 2017, 37(10):1140-1150 Hu X G, Wei Z, Mu L. Graphene oxide nanosheets at trace concentrations elicit neurotoxicity in the offspring of zebrafish[J]. Carbon, 2017, 117:182-191 Shah S A, Yoon G H, Ahmad A, et al. Nanoscale-alumina induces oxidative stress and accelerates amyloid beta (Aβ) production in ICR female mice[J]. Nanoscale, 2015, 7(37):15225-15237 Zhang L L, Bai R, Li B, et al. Rutile TiO2 particles exert size and surface coating dependent retention and lesions on the murine brain[J]. Toxicology Letters, 2011, 207(1):73-81 Wu J, Wang C, Sun J, et al. Neurotoxicity of silica nanoparticles:Brain localization and dopaminergic neurons damage pathways[J]. ACS Nano, 2011, 5(6):4476-4489 Neves A R, Queiroz J F, Lima S A C, et al. Apo E-functionalization of solid lipid nanoparticles enhances brain drug delivery:Uptake mechanism and transport pathways[J]. Bioconjugate Chemistry, 2017, 28(4):995-1004 Mendonça M C, Soares E S, de Jesus M B, et al. Reduced graphene oxide induces transient blood-brain barrier opening:An in vivo study[J]. Journal of Nanobiotechnology, 2015, 13:78 Liu X, Sui B Y, Sun J. Blood-brain barrier dysfunction induced by silica NPs in vitro and in vivo:Involvement of oxidative stress and Rho-kinase/JNK signaling pathways[J]. Biomaterials, 2017, 121:64-82 Kafa H, Wang J T, Rubio N, et al. Translocation of LRP1 targeted carbon nanotubes of different diameters across the blood-brain barrier in vitro and in vivo[J]. Journal of Controlled Release:Official Journal of the Controlled Release Society, 2016, 225:217-229 Huang R Q, Ke W L, Han L, et al. Brain-targeting mechanisms of lactoferrin-modified DNA-loaded nanoparticles[J]. Journal of Cerebral Blood Flow and Metabolism:Official Journal of the International Society of Cerebral Blood Flow and Metabolism, 2009, 29(12):1914-1923 Quevedo A C, Iseult L, Eugenia V. Mechanisms of silver nanoparticle uptake by embryonic zebrafish cells[J]. Nanomaterials, 2021, 11(10):2699 Kumari M, Rajak S, Singh S P, et al. Repeated oral dose toxicity of iron oxide nanoparticles:Biochemical and histopathological alterations in different tissues of rats[J]. Journal of Nanoscience and Nanotechnology, 2012, 12(3):2149-2159 Hong F S, Sheng L, Ze Y G, et al. Suppression of neurite outgrowth of primary cultured hippocampal neurons is involved in impairment of glutamate metabolism and NMDA receptor function caused by nanoparticulate TiO2[J]. Biomaterials, 2015, 53:76-85 Guo D D, Bi H S, Wang D G, et al. Zinc oxide nanoparticles decrease the expression and activity of plasma membrane calcium ATPase, disrupt the intracellular calcium homeostasis in rat retinal ganglion cells[J]. The International Journal of Biochemistry & Cell Biology, 2013, 45(8):1849-1859 Setyawati M I, Yuan X, Xie J P, et al. The influence of lysosomal stability of silver nanomaterials on their toxicity to human cells[J]. Biomaterials, 2014, 35(25):6707-6715 Mao B H, Tsai J C, Chen C W, et al. Mechanisms of silver nanoparticle-induced toxicity and important role of autophagy[J]. Nanotoxicology, 2016, 10(8):1021-1040 Fu P P, Xia Q S, Hwang H M, et al. Mechanisms of nanotoxicity:Generation of reactive oxygen species[J]. Journal of Food and Drug Analysis, 2014, 22(1):64-75 Phenrat T, Long T C, Lowry G V, et al. Partial oxidation ("aging") and surface modification decrease the toxicity of nanosized zerovalent iron[J]. Environmental Science & Technology, 2009, 43(1):195-200 Long T C, Saleh N, Tilton R D, et al. Titanium dioxide (P25) produces reactive oxygen species in immortalized brain microglia (BV2):Implications for nanoparticle neurotoxicity[J]. Environmental Science & Technology, 2006, 40(14):4346-4352 Huerta-García E, Pérez-Arizti J A, Márquez-Ramírez S G, et al. Titanium dioxide nanoparticles induce strong oxidative stress and mitochondrial damage in glial cells[J]. Free Radical Biology & Medicine, 2014, 73:84-94 Costa C S, Ronconi J V, Daufenbach J F, et al. In vitro effects of silver nanoparticles on the mitochondrial respiratory chain[J]. Molecular and Cellular Biochemistry, 2010, 342(1-2):51-56 Ziemińska E, Stafiej A, Strużyńska L. The role of the glutamatergic NMDA receptor in nanosilver-evoked neurotoxicity in primary cultures of cerebellar granule cells[J]. Toxicology, 2014, 315:38-48 Xie H J, Wu J. Silica nanoparticles induce alpha-synuclein induction and aggregation in PC12-cells[J]. Chemico-Biological Interactions, 2016, 258:197-204 Geppert M, Hohnholt M C, Nürnberger S, et al. Ferritin up-regulation and transient ROS production in cultured brain astrocytes after loading with iron oxide nanoparticles[J]. Acta Biomaterialia, 2012, 8(10):3832-3839 Chen Z W, Yin J J, Zhou Y T, et al. Dual enzyme-like activities of iron oxide nanoparticles and their implication for diminishing cytotoxicity[J]. ACS Nano, 2012, 6(5):4001-4012 Stern S T, Adiseshaiah P P, Crist R M. Autophagy and lysosomal dysfunction as emerging mechanisms of nanomaterial toxicity[J]. Particle and Fibre Toxicology, 2012, 9:20 Luther E M, Petters C, Bulcke F, et al. Endocytotic uptake of iron oxide nanoparticles by cultured brain microglial cells[J]. Acta Biomaterialia, 2013, 9(9):8454-8465 Hsiao I L, Chang C C, Wu C Y, et al. Indirect effects of TiO2 nanoparticle on neuron-glial cell interactions[J]. Chemico-Biological Interactions, 2016, 254:34-44 Moquin A, Hutter E, Choi A O, et al. Caspase-1 activity in microglia stimulated by pro-inflammagen nanocrystals[J]. ACS Nano, 2013, 7(11):9585-9598 Ye D, Raghnaill M N, Bramini M, et al. Nanoparticle accumulation and transcytosis in brain endothelial cell layers[J]. Nanoscale, 2013, 5(22):11153-11165 Erriquez J, Bolis V, Morel S, et al. Nanosized TiO2 is internalized by dorsal root ganglion cells and causes damage via apoptosis[J]. Nanomedicine:Nanotechnology, Biology, and Medicine, 2015, 11(6):1309-1319 Petters C, Thiel K, Dringen R. Lysosomal iron liberation is responsible for the vulnerability of brain microglial cells to iron oxide nanoparticles:Comparison with neurons and astrocytes[J]. Nanotoxicology, 2016, 10(3):332-342 Ze Y G, Sheng L, Zhao X Y, et al. TiO2 nanoparticles induced hippocampal neuroinflammation in mice[J]. PLoS One, 2014, 9(3):e92230 El-Ghor A A, Noshy M M, Galal A, et al. Normalization of nano-sized TiO2-induced clastogenicity, genotoxicity and mutagenicity by chlorophyllin administration in mice brain, liver, and bone marrow cells[J]. Toxicological Sciences:An Official Journal of the Society of Toxicology, 2014, 142(1):21-32 Asharani P, Sethu S, Lim H K, et al. Differential regulation of intracellular factors mediating cell cycle, DNA repair and inflammation following exposure to silver nanoparticles in human cells[J]. Genome Integrity, 2012, 3(1):2 Verano-Braga T, Miethling-Graff R, Wojdyla K, et al. Insights into the cellular response triggered by silver nanoparticles using quantitative proteomics[J]. ACS Nano, 2014, 8(3):2161-2175 He K Y, Liang X, Wei T T, et al. DNA damage in BV-2 cells:An important supplement to the neurotoxicity of CdTe quantum dots[J]. Journal of Applied Toxicology, 2019, 39(3):525-539 Valdiglesias V, Costa C, Kiliç G, et al. Neuronal cytotoxicity and genotoxicity induced by zinc oxide nanoparticles[J]. Environment International, 2013, 55:92-100 Valdiglesias V, Costa C, Sharma V, et al. Comparative study on effects of two different types of titanium dioxide nanoparticles on human neuronal cells[J]. Food and Chemical Toxicology, 2013, 57:352-361 Wang J T, Deng X B, Zhang F, et al. ZnO nanoparticle-induced oxidative stress triggers apoptosis by activating JNK signaling pathway in cultured primary astrocytes[J]. Nanoscale Research Letters, 2014, 9(1):117 Zhang R, Piao M J, Kim K C, et al. Endoplasmic reticulum stress signaling is involved in silver nanoparticles-induced apoptosis[J]. The International Journal of Biochemistry & Cell Biology, 2012, 44(1):224-232 Simard J C, Vallieres F, de Liz R, et al. Silver nanoparticles induce degradation of the endoplasmic reticulum stress sensor activating transcription factor-6 leading to activation of the NLRP-3 inflammasome[J]. The Journal of Biological Chemistry, 2015, 290(9):5926-5939 Zhang Q L, Li M Q, Ji J W, et al. In vivo toxicity of nano-alumina on mice neurobehavioral profiles and the potential mechanisms[J]. International Journal of Immunopathology and Pharmacology, 2011, 24(1 Suppl.):23S-29S Sun C, Yin N Y, Wen R X, et al. Silver nanoparticles induced neurotoxicity through oxidative stress in rat cerebral astrocytes is distinct from the effects of silver ions[J]. Neurotoxicology, 2016, 52:210-221 Song B, Zhang Y L, Liu J, et al. Unraveling the neurotoxicity of titanium dioxide nanoparticles:Focusing on molecular mechanisms[J]. Beilstein Journal of Nanotechnology, 2016, 7:645-654 Li J J, Hartono D, Ong C N, et al. Autophagy and oxidative stress associated with gold nanoparticles[J]. Biomaterials, 2010, 31(23):5996-6003 Halamoda Kenzaoui B, Chapuis Bernasconi C, Guney-Ayra S, et al. Induction of oxidative stress, lysosome activation and autophagy by nanoparticles in human brain-derived endothelial cells[J]. The Biochemical Journal, 2012, 441(3):813-821 Gong C M, Tao G H, Yang L Q, et al. Methylation of PARP-1 promoter involved in the regulation of nano-SiO2-induced decrease of PARP-1 mRNA expression[J]. Toxicology Letters, 2012, 209(3):264-269 Gong C M, Tao G H, Yang L Q, et al. SiO2 nanoparticles induce global genomic hypomethylation in HaCaT cells[J]. Biochemical and Biophysical Research Communications, 2010, 397(3):397-400 Chen Y, Xu M, Zhang J, et al. Genome-wide DNA methylation variations upon exposure to engineered nanomaterials and their implications in nanosafety assessment[J]. Advanced Materials, 2017, 29(6):1604580 -
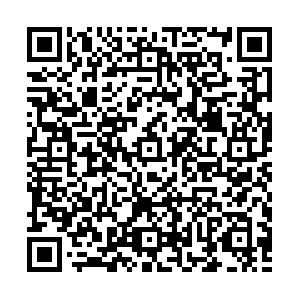
计量
- 文章访问数: 2226
- HTML全文浏览数: 2226
- PDF下载数: 108
- 施引文献: 0