重金属影响中枢神经系统的微生物-肠-脑轴途径
Influences of heavy metal on the central nervous system through microbe-gut-brain axis pathway
-
摘要: 人类生产活动排放出的过量重金属直接危害人体健康.目前,有关重金属诱导中枢神经系统疾病发生的研究受到越来越多的关注,经胃肠道吸收是重金属进入人体的主要途径之一,而肠道作为人体内最大的微生态系统,对维持神经系统的正常功能具有重要的生物作用.本文对表征肠道微生物多样性改变的测序方法,以及环境中广泛存在的重金属锰(Mn)、铅(Pb)、镉(Cd)、砷(As)通过影响微生物-肠-脑轴造成神经系统疾病的潜在机制进行总结,并对重金属等污染物基于肠道微生物引发神经系统疾病的预防和治疗进行展望.Abstract: Human production activities release excessive heavy metals and directly harm human health. At present, the researches on the heavy metal-induced central nervous system diseases has received increasing attention. Absorption through the gastrointestinal tract is one of the major pathways for the entry of heavy metals into the human body. The intestine is the largest micro-ecological system in the human body, which plays an important biological role in maintaining the normal functions of the nervous system. This study describes the sequencing method for characterizing the diversity of intestinal microbes, and summarizes the potential mechanisms of the microbe-gut-brain axis effects on the neurological diseases caused by the widespread presence of heavy metals, including manganese (Mn), lead (Pb), cadmium (Cd), and arsenic (As) in the environment. We will provide the prevention and treatment of heavy metals-induced neurological diseases based on intestinal microbes.
-
Key words:
- heavy metals /
- intestinal microbes /
- central nervous system /
- microbe-gut-brain axis
-
-
[1] MANARY M J. Human gut microbiome viewed across age and geography[J]. Nature, 2012, 486(7402):222-227. [2] LEY R E, PETERSON DA, GORDON J I. Ecological and evolutionary forces shaping microbial diversity in the human intestine[J]. Cell, 2006, 124(4):837-848. [3] GILL S R, POP M, DEBOY R T, et al. Metagenomic analysis of the human distal gut microbiome[J]. Science, 2006, 312(5778):1355-1359. [4] YOUNG V B, BRITTON R A, SCHMIDT T M. The human microbiome and infectious diseases:Beyond koch[J]. Interdisciplinary Perspectives on Infectious Diseases, 2009, 2008:296873. [5] SANDLER RH, FINEGOLD SM, BOLTE ER, et al. Short-term benefit from oral vancomycin treatment of regressive-onset autism[J]. Journal of Child Neurology, 2000, 15(7):429-435. [6] MULAK A, BONAZ B. Brain-gut-microbiota axis in Parkinson's disease[J]. World Journal of Gastroenterology, 2015, 21(37):10609-10620. [7] OLANOW CW, WAKEMAN DR, KORDOWER JH. Peripheral alpha-synuclein and Parkinson's disease[J]. Movement Disorders Official Journal of the Movement Disorder Society, 2014, 29(8):963-966. [8] MONACHESE M, BURTON JP, REID G. Bioremediation and tolerance of humans to heavy metals through microbial processes:a potential role for probiotics?[J]. Applied & Environmental Microbiology, 2012, 78(18):6397-6404. [9] ZLATKO Z, BILBAN M, FABJAN T, et al. Lead exposure and oxidative stress in coal miners[J]. Biomedical and Environmental Sciences, 2017, 30(11):841-845. [10] CHEN, RONG, WANG, et al. The pollution character analysis and risk assessment for metals in dust and PM10 around road from China[J]. Biomedical and Environmental Sciences, 2015, 28(1):44-56. [11] REANEY SH, BENCH G, SMITH DR. Brain Accumulation and Toxicity of Mn(Ⅱ) and Mn(Ⅲ) Exposures[J]. Toxicological Sciences An Official Journal of the Society of Toxicology, 2006, 93(1):114-124. [12] ATTAR AM, KHARKHANEH A, ETEMADIFAR M, et al. Serum mercury level and multiple sclerosis[J]. Biological Trace Element Research, 2012, 146(2):150-153. [13] OLSON ND, LUND SP, ZOOK JM, et al. International interlaboratory study comparing single organism 16S rRNA gene sequencing data:beyond consensus sequence comparisons[J]. Biomolecular Detection & Quantification, 2015, 3:17-24. [14] WANG Q, GARRITY GM, TIEDJE JM, et al. Naïve bayesian classifier for rapid assignment of rRNA sequences into the new bacterial taxonomy[J]. Applied & Environmental Microbiology, 2007, 73(16):5261-5267. [15] PATEL J B. 16S rRNA gene sequencing for bacterial pathogen identification in the clinical laboratory[J]. Mol Diagn, 2001, 6(4):313-321. [16] HANDELSMAN J, RONDON MR, BRADY SF, et al. Molecular biological access to the chemistry of unknown soil microbes:A new frontier for natural products[J]. Chem Biol, 1998, 5(10):R245-249. [17] 夏照华, 何建行. 宏基因组测序在传染性疾病中的研究进展[J]. 中国微生态学杂志, 2017, 29(2):245-248. XIA Z H,HE J X. Research progress of metagenomic sequencing in infectious diseases[J]. Chinese Journal of Microecology, 2017, 29(2):245-248(in Chinese).
[18] BIKEL S, VALDEZ-LARA A, CORNEJO-GRANADOS F, et al. Combining metagenomics, metatranscriptomics and viromics to explore novel microbial interactions:Towards a systems-level understanding of human microbiome[J]. Computational & Structural Biotechnology Journal, 2015, 13:390-401. [19] DAVIDSON R M, EPPERSON L E. Microbiome sequencing methods for studying human diseases[J]. Methods Mol Biol, 2018, 1706:77-90. [20] CHI L, BIAN X, GAO B, et al. The effects of an environmentally relevant level of arsenic on the gut microbiome and its functional metagenome[J]. Toxicological Sciences An Official Journal of the Society of Toxicology, 2017, 160(2):193-204. [21] LIANG C, BIAN X, BEI G, et al. Sex-specific effects of arsenic exposure on the trajectory and function of the gut microbiome[J]. Chemical Research in Toxicology, 2016, 29(6):949-951. [22] MILATOVIC D, RC ZMS. Oxidative damage and neurodegeneration in manganese-induced neurotoxicity[J]. Toxicology & Applied Pharmacology, 2009, 240(2):219-225. [23] GUNTER TE, GAVIN CE, ASCHNER M, et al. Speciation of manganese in cells and mitochondria:A search for the proximal cause of manganese neurotoxicity[J]. Neurotoxicology, 2006, 27(5):765-776. [24] KOMATSU F, KAGAWA Y, KAWABATA T, et al. A high accumulation of hair minerals in mongolian people:2(nd) report; influence of manganese, iron, lead, cadmium and aluminum to oxidative stress, Parkinsonism and arthritis[J]. Current Aging Science, 2011, 4(1):42-56. [25] LIANG C, BEI G, BIAN X, et al. Manganese-induced sex-specific gut microbiome perturbations in C57BL/6 mice[J]. Toxicology & Applied Pharmacology, 2017, 331:142-153. [26] LU K, ABO RP, SCHLIEPER KA, et al. Arsenic exposure perturbs the gut microbiome and its metabolic profile in mice:An integrated metagenomics and metabolomics analysis[J]. Environmental Health Perspectives, 2014, 122(3):284-291. [27] JIN Y, WU S, ZENG Z, et al. Effects of environmental pollutants on gut microbiota[J]. Environmental Pollution, 2017, 222:1-9. [28] KOENIG JE, KLAENHAMMER TR. Succession of microbial consortia in the developing infant gut microbiome[J]. Proceedings of the National Academy of Science, 2011, 108(Suppl 1):4578-4585. [29] LEBLANC J G, MILANI C, DE GIORI G S, et al. Bacteria as vitamin suppliers to their host:A gut microbiota perspective[J]. Curr Opin Biotechnol, 2013, 24(2):160-168. [30] IANNIELLO R G, RICCIARDI A, PARENTE E, et al. Aeration and supplementation with heme and menaquinone affect survival to stresses and antioxidant capability of lactobacillus casei strains[J]. LWT-Food Science and Technology, 2015, 60(2):817-824. [31] FLORA SJS, MITTAL M, MISHRA D. Co-exposure to arsenic and fluoride on oxidative stress, glutathione linked enzymes, biogenic amines and DNA damage in mouse brain[J]. Journal of the Neurological Sciences, 2009, 285(1-2):198-205. [32] CANI P D, BIBILONI R, KNAUF C, et al. Changes in gut microbiota control metabolic endotoxemia-induced inflammation in high-fat diet-induced obesity and diabetes in mice[J]. Diabetes, 2008, 57(6):1470-1481. [33] HOOPER L V, DAN R L, MACPHERSON A J. Interactions between the microbiota and the immune system[J]. Science, 2012, 336(6086):1268-1273. [34] MICHEL O, GINANNI R, DUCHATEAU J, et al. Domestic endotoxin exposure and clinical severity of asthma[J]. Clinical & Experimental Allergy, 1991, 21(4):441-448. [35] ZHU J P, WU K, LI J Y, et al. Cryptoporus volvatus polysaccharides attenuate LPS-induced expression of pro-inflammatory factors via the TLR2 signaling pathway in human alveolar epithelial cells[J]. Pharmaceutical Biology, 2016, 54(2):347-353. [36] MEDZHITOV R. Medzhitov R. Recognition of microorganisms and activation of the immune response.[J]. Nature, 2007, 449(7164):819-826. [37] CRYAN J F, DINAN T G. Mind-altering microorganisms:The impact of the gut microbiota on brain and behaviour[J]. Nature Reviews Neuroscience, 2012, 13(10):701-712. [38] ROCHFORT K D, CUMMINS P M. The blood-brain barrier endothelium:a target for pro-inflammatory cytokines[J]. Biochemical Society Transactions, 2015, 43(4):702-706. [39] DANTZER R, KONSMAN J P, BLUTHé R M, et al. Neural and humoral pathways of communication from the immune system to the brain:parallel or convergent?[J]. Autonomic Neuroscience Basic & Clinical, 2000, 85(1):60-65. [40] QUAN N, WHITESIDE M, HERKENHAM M. Time course and localization patterns of interleukin-1beta messenger RNA expression in brain and pituitary after peripheral administration of lipopolysaccharide[J]. Neuroscience, 1998, 83(1):281-293. [41] KONSMAN JP, VIGUES S, MACKERLOVA L, et al. Rat brain vascular distribution of interleukin-1 type-1 receptor immunoreactivity:relationship to patterns of inducible cyclooxygenase expression by peripheral inflammatory stimuli[J]. Journal of Comparative Neurology, 2004, 472(1):113-129. [42] BUHNIK-ROSENBLAU K, MOSHE-BELIZOWSKI S, DANIN-POLEG Y, et al. Genetic modification of iron metabolism in mice affects the gut microbiota[J]. Biometals, 2012, 25(5):883-892. [43] ZHENG W, ZHAO Q, SLAVKOVICH V, et al. Alteration of iron homeostasis following chronic exposure to manganese in rats[J]. Brain Research, 1999, 833(1):125-132. [44] LE W. Role of iron in UPS impairment model of Parkinson's disease[J]. Parkinsonism & Related Disorders, 2014, 20(Suppl 1):S158. [45] CHUA ACG, MORGAN E H. Effects of iron deficiency and iron overload on manganese uptake and deposition in the brain and other organs of the rat[J]. Biological Trace Element Research, 1996, 55(1-2):39-54. [46] ROTH J A, GARRICK M D. Iron interactions and other biological reactions mediating the physiological and toxic actions of manganese[J]. Biochemical Pharmacology, 2003, 66(1):1-13. [47] CROSSGROVE J, ZHENG W. Manganese toxicity upon overexposure[J]. Nmr in Biomedicine, 2004, 17(8):544-553. [48] JONES BV, BEGLEY M, HILL C, et al. Functional and comparative metagenomic analysis of bile salt hydrolase activity in the human gut microbiome[J]. Proc Natl Acad Sci U S A, 2008, 105(36):13580-13585. [49] GRIFFITHS W J, SJöVALL J. Bile acids:analysis in biological fluids and tissues[J]. Journal of Lipid Research, 2010, 51(1):23-41. [50] GAO B, CHI L, MAHBUB R, et al. Multi-omics reveals that lead exposure disturbs gut microbiome development, key metabolites and metabolic pathways[J]. Chemical Research in Toxicology, 2017, 30(4):996-1005. [51] DIETSCH J M, TURLEY S D. Cholesterol metabolism in the central nervous system during early development and in the mature animal[J]. Journal of Lipid Research, 2004, 45(8):1375-1397. [52] YADAV R S, TIWARI N K. Lipid Integration in neurodegeneration:an overview of Alzheimer's disease[J]. Molecular Neurobiology, 2014, 50(1):168-176. [53] ANTHARAM V C, MCEWEN D C, GARRETT T J, et al. An integrated metabolomic and microbiome analysis identified specific gut microbiota associated with fecal cholesterol and coprostanol in clostridium difficile Infection[J]. Plos One, 2016, 11(2):e0148824. [54] HAENEN D, ZHANG J, SOUZA DA SILVA C, et al. A diet high in resistant starch modulates microbiota composition, SCFA concentrations, and gene expression in pig intestine[J]. Journal of Nutrition, 2013, 143(3):274-283. [55] WONG J M, DE S R, KENDALL C W, et al. Colonic health:Fermentation and short chain fatty acids[J]. Journal of Clinical Gastroenterology, 2006, 40(3):235-243. [56] TAN J, MCKENZIE C, POTAMITIS M, et al. The role of short-chain fatty acids in health and disease[J]. Advances in Immunology, 2014, 121(January):91-119. [57] BRANISTE V, ALASMAKH M, KOWAL C, et al. The gut microbiota influences blood-brain barrier permeability in mice[J]. Science Translational Medicine, 2014, 6(263):263ra158. [58] DE THEIJE C G, WOPEREIS H, RAMADAN M, et al. Altered gut microbiota and activity in a murine model of autism spectrum disorders[J]. Brain Behavior & Immunity, 2014, 37(3):197-206. [59] SAMPSON T R, DEBELIUS J W, THRON T, et al. Gut microbiota regulate motor deficits and neuroinflammation in a model of Parkinson's disease[J]. Cell, 2016, 167(6):1469-1480. [60] ZHANG S, JIN Y, ZENG Z, et al. Subchronic exposure of mice to cadmium perturbs their hepatic energy metabolism and gut microbiome[J]. Chemical Research in Toxicology, 2015, 28(10):2000-2009. [61] LIU Y, LI Y, LIU K, et al. Exposing to cadmium stress cause profound toxic effect on microbiota of the mice intestinal tract[J]. Plos One, 2014, 9(2):e85323. [62] FROST G, SLEETH M L, SAHURIARISOYLU M, et al. The short-chain fatty acid acetate reduces appetite via a central homeostatic mechanism[J]. Nature Communications, 2014, 5:3611. [63] WU J, WEN X W, FAULK C, et al. Perinatal lead exposure alters gut microbiota composition and results in sex-specific bodyweight increases in adult mice[J]. Toxicological Sciences, 2016, 151(2):324-333. [64] BARANOWSKABOSIACKA I, FALKOWSKA A, GUTOWSKA I, et al. Glycogen metabolism in brain and neurons-astrocytes metabolic cooperation can be altered by pre-and neonatal lead (Pb) exposure[J]. Toxicology, 2017, 390:146-158. [65] FRYER K L, BROWN A M. Pluralistic roles for glycogen in the central and peripheral nervous systems[J]. Metabolic Brain Disease, 2015, 30(1):299-306. [66] LYTE M. Microbial endocrinology in the microbiome-gut-brain axis:how bacterial production and utilization of neurochemicals influence behavior[J]. PLOS Pathogens,9,11(2013-11-14), 2013, 9(11):e1003726. [67] MORTON G J, SCHWARTZ M W. Leptin and the CNS control of glucose metabolism[J]. Physiological Reviews, 2011, 91(2):389-411. [68] BIAGIOLI M, PIFFERI S, RAGGHIANTI M, et al. Endoplasmic reticulum stress and alteration in calcium homeostasis are involved in cadmium-induced apoptosis[J]. Cell Calcium, 2008, 43(2):184-195. [69] SHI W, GUAN X, HAN Y, et al. Waterborne Cd2+ weakens the immune responses of blood clam through impacting Ca2+ signaling and Ca2+ related apoptosis pathways[J]. Fish & Shellfish Immunology, 2018, 77:208-213. [70] 许运智, 黄锁义. 钙和人体健康[J]. 化学教学, 2003, (12):28-29. XU Y Z,HUANG S Y. Calcium and human health[J]. Chemistry Teaching, 2003 , (12):28-29(in Chinese).
[71] NISHIMURA Y, YAMAGUCHI J Y, KANADA A, et al. Increase in intracellular Cd2+ concentration of rat cerebellar granule neurons incubated with cadmium chloride:Cadmium cytotoxicity under external Ca2+ -free condition[J]. Toxicology in Vitro, 2006, 20(2):211-216. [72] YUAN Y, JIANG CY, XU H, et al. Cadmium-induced apoptosis in primary rat cerebral cortical neurons culture is mediated by a calcium signaling pathway[J]. Plos One, 2013, 8(5):e64330. [73] MIN K S, SANO E, UEDA H, et al. Dietary deficiency of calcium and/or iron, an age-related risk factor for renal accumulation of cadmium in mice[J]. Biological & Pharmaceutical Bulletin, 2015, 38(10):1557-1563. [74] YANG X, WEN G, TUO B, et al. Molecular mechanisms of calcium signaling in the modulation of small intestinal ion transports and bicarbonate secretion[J]. Oncotarget, 2018, 9(3):3727-3740. [75] FURNESS J B, CALLAGHAN B P, RIVERA L R, et al. The enteric nervous system and gastrointestinal innervation:integrated local and central control[M]. New York:Springer New York, 2014. [76] SHARON G, GARG N, DEBELIUS J, et al. Specialized metabolites from the microbiome in health and disease[J]. Cell Metabolism, 2014, 20(5):719-730. [77] BARRETT E, ROSS R P, O'TOOLE P W, et al. γ-Aminobutyric acid production by culturable bacteria from the human intestine[J]. Journal of Applied Microbiology, 2012, 113(2):411-417. [78] BRAVO J A, FORSYTHE P, CHEW M V, et al. Ingestion of lactobacillus strain regulates emotional behavior and central GABA receptor expression in a mouse via the vagus nerve[J]. Proceedings of the National Academy of Sciences of the United States of America, 2011, 108(38):16050-16055. [79] SEQUERRA E B, GARDINO P, HEDIN-PEREIRA C, et al. Putrescine as an important source of GABA in the postnatal rat subventricular zone[J]. Neuroscience, 2007, 146(2):489-493. [80] POULTER M O, SINGHAL R, BROWN L A, et al. GABA(A) receptor subunit messenger RNA expression in the enteric nervous system of the rat:implications for functional diversity of enteric GABA(A) receptors[J]. Neuroscience, 1999, 93(3):1159-1165. [81] FERNSTROM J D. Tryptophan availability and serotonin synthesis in brain[M]. Berlin:Springer Berlin Heidelberg, 1988. [82] GERSHON M D, TACK J. The serotonin signaling system:from basic understanding to drug development for functional GI disorders[J]. Gastroenterology, 2007, 132(1):397-414. [83] FOSTER J A, RINAMAN L, CRYAN J F. Stress & the gut-brain axis:Regulation by the microbiome[J]. Neurobiol Stress, 2017, 7:124-136. [84] SANG H R, POTHOULAKIS C, MAYER E A. Principles and clinical implications of the brain-gut-enteric microbiota axis[J]. Nature Reviews Gastroenterology & Hepatology, 2009, 6(5):306-314. [85] NICHOLSON J K, HOLMES E, KINROSS J, et al. Host-gut microbiota metabolic interactions.[J]. Science, 2012, 336(6086):1262-1267. [86] CLAVEL T, GOMES-NETO J C, LAGKOUVARDOS I, et al. Deciphering interactions between the gut microbiota and the immune system via microbial cultivation and minimal microbiomes[J]. Immunological Reviews, 2017, 279(1):8-22. [87] SINHA V, MISHRA R, KUMAR A, et al. Amplification of arsH gene in lactobacillus acidophilus resistant to arsenite[J]. Biotechnology, 2011, 10(1):101-107. [88] ROBINSON J B, TUOVINEN O H. Mechanisms of microbial resistance and detoxification of mercury and organomercury compounds:physiological, biochemical, and genetic analyses[J]. Microbiol Rev, 1984, 48(2):95-124. [89] OSBORN A M, BRUCE K D, STRIKE P, et al. Distribution, diversity and evolution of the bacterial mercury resistance (mer) operon[J]. Fems Microbiology Reviews, 1997, 19(4):239-262. [90] KRANENBURG R V, GOLIC N, BONGERS R, et al. Functional analysis of three plasmids from lactobacillus plantarum[J]. Applied & Environmental Microbiology, 2005, 71(3):1223-1230. [91] TABATADZE T, ZHORZHOLIANI L, KHERKHEULIDZE M, et al. Hair heavy metal and essential trace element concentration in children with autism spectrum disorder[J]. Georgian Medical News, 2015(248):77-82. [92] ALBIZZATI A, MORÃ L, DI CANDIA D, et al. Normal concentrations of heavy metals in autistic spectrum disorders[J]. Minerva Pediatrica, 2012, 64(1):27-31. [93] ALESSANDRA M, MARTINA L, FRANCESCO F, et al. Environment, dysbiosis, immunity and sex-specific susceptibility:A translational hypothesis for regressive autism pathogenesis[J]. Nutritional Neuroscience, 2015, 18(4):145-161. [94] FINEGOLD S M. Therapy and epidemiology of autism-Clostridial spores as key elements[J]. Medical Hypotheses, 2008, 70(3):508-511. [95] TILLISCH K, LABUS J, KILPATRICK L, et al. Consumption of fermented milk product with probiotic modulates brain activity[J]. Gastroenterology, 2013, 144(7):1394-1401. -
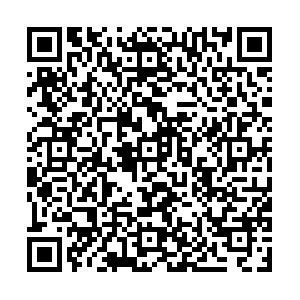
计量
- 文章访问数: 2022
- HTML全文浏览数: 1994
- PDF下载数: 77
- 施引文献: 0