饮用水中新型环状消毒副产物的毒性研究进展
Research Progress on Toxicity of New Cyclic Disinfection Byproducts in Drinking Water
-
摘要: 饮用水中新型环状消毒副产物(disinfection byproducts,DBPs)因具有较高的生物毒性,受到越来越广泛的关注。本文简要概述了国内外饮用水中新型环状DBPs检测情况及暴露水平,重点探讨了新型环状DBPs的细胞毒性、遗传毒性和内分泌干扰效应,并总结了定量构效关系(quantitative structure-activity relationship,QSAR)在毒性效应预测方面的应用,旨在为新型环状DBPs的毒性及致毒机理研究提供理论参考。Abstract: New cyclic disinfection byproducts (DBPs) in drinking water have been intensively attracting concerns due to their significantly greater toxicity than commonly known DBPs. This paper briefly reviewed the detection and occurrence of new cyclic DBPs in drinking water, and discussed cytotoxicity, genotoxicity, and endocrine disrupting effect of these new cyclic DBPs in detail. The application of quantitative structure-activity relationship (QSAR) in toxicity prediction was also summarized. This review aimed to provide a theoretical reference for further toxicity and mechanism studies of new cyclic DBPs.
-
Key words:
- new cyclic disinfection byproducts /
- drinking water /
- toxicity /
- QSAR
-
-
Calderon R L. The epidemiology of chemical contaminants of drinking water[J]. Food and Chemical Toxicology, 2000, 38:S13-S20 Grünwald A, Št'astný B, Slavíčková K, et al. Formation of haloforms during chlorination of natural waters[J]. Acta Polytechnica, 2002, 42(2):234-243 Richardson S D. Water analysis:Emerging contaminants and current issues[J]. Analytical Chemistry, 2007, 79(12):4295-4323 Wagner E D, Plewa M J. CHO cell cytotoxicity and genotoxicity analyses of disinfection by-products:An updated review[J]. Journal of Environmental Sciences, 2017, 58:64-76 Grellier J, Rushton L, Briggs D J, et al. Assessing the human health impacts of exposure to disinfection by-products-A critical review of concepts and methods[J]. Environment International, 2015, 78:61-81 Wright J M, Evans A, Kaufman J A, et al. Disinfection by-product exposures and the risk of specific cardiac birth defects[J]. Environmental Health Perspectives, 2017, 125(2):269-277 Regli S, Chen J, Messner M, et al. Estimating potential increased bladder cancer risk due to increased bromide concentrations in sources of disinfected drinking waters[J]. Environmental Science & Technology, 2015, 49(22):13094-13102 Diana M, Felipe-Sotelo M, Bond T. Disinfection byproducts potentially responsible for the association between chlorinated drinking water and bladder cancer:A review[J]. Water Research, 2019, 162:492-504 Villanueva C M, Kogevinas M, Cordier S, et al. Assessing exposure and health consequences of chemicals in drinking water:Current state of knowledge and research needs[J]. Environmental Health Perspectives, 2014, 122(3):213-221 King W D, Marrett L D. Case-control study of bladder cancer and chlorination by-products in treated water (Ontario, Canada)[J]. Cancer Causes & Control, 1996, 7(6):596-604 Villanueva C M, Cantor K P, Cordier S, et al. Disinfection byproducts and bladder cancer:A pooled analysis[J]. Epidemiology, 2004, 15(3):357-367 Richardson S D, Plewa M J, Wagner E D, et al. Occurrence, genotoxicity, and carcinogenicity of regulated and emerging disinfection by-products in drinking water:A review and roadmap for research[J]. Mutation Research/Reviews in Mutation Research, 2007, 636(1-3):178-242 Krasner S W, Weinberg H S, Richardson S D, et al. Occurrence of a new generation of disinfection byproducts[J]. Environmental Science & Technology, 2006, 40(23):7175-7185 Zhai H Y, Zhang X R. Formation and decomposition of new and unknown polar brominated disinfection byproducts during chlorination[J]. Environmental Science & Technology, 2011, 45(6):2194-2201 Zhai H Y, Zhang X R, Zhu X H, et al. Formation of brominated disinfection byproducts during chloramination of drinking water:New polar species and overall kinetics[J]. Environmental Science & Technology, 2014, 48(5):2579-2588 Gong T T, Tao Y X, Zhang X R, et al. Transformation among aromatic iodinated disinfection byproducts in the presence of monochloramine:From monoiodophenol to triiodophenol and diiodonitrophenol[J]. Environmental Science & Technology, 2017, 51(18):10562-10571 Hu S Y, Gong T T, Zhu H T, et al. Formation and decomposition of new iodinated halobenzoquinones during chloramination in drinking water[J]. Environmental Science & Technology, 2020, 54(8):5237-5248 Pan Y, Wang Y, Li A M, et al. Detection, formation and occurrence of 13 new polar phenolic chlorinated and brominated disinfection byproducts in drinking water[J]. Water Research, 2017, 112:129-136 Huang Y, Li H, Zhou Q, et al. New phenolic halogenated disinfection byproducts in simulated chlorinated drinking water:Identification, decomposition, and control by ozone-activated carbon treatment[J]. Water Research, 2018, 146:298-306 Qin F, Zhao Y Y, Zhao Y L, et al. A toxic disinfection by-product, 2,6-dichloro-1,4-benzoquinone, identified in drinking water[J]. Angewandte Chemie, 2010, 49(4):790-792 Pan Y, Zhang X R. Four groups of new aromatic halogenated disinfection byproducts:Effect of bromide concentration on their formation and speciation in chlorinated drinking water[J]. Environmental Science & Technology, 2013, 47(3):1265-1273 Zhao Y L, Qin F, Boyd J M, et al. Characterization and determination of chloro- and bromo-benzoquinones as new chlorination disinfection byproducts in drinking water[J]. Analytical Chemistry, 2010, 82(11):4599-4605 Sayess R, Khalil A, Shah M, et al. Comparative cytotoxicity of six iodinated disinfection byproducts on nontransformed epithelial human colon cells[J]. Environmental Science & Technology Letters, 2017, 4(4):143-148 Plewa M J, Wagner E D, Richardson S D, et al. Chemical and biological characterization of newly discovered iodoacid drinking water disinfection byproducts[J]. Environmental Science & Technology, 2004, 38(18):4713-4722 Plewa M J, Simmons J E, Richardson S D, et al. Mammalian cell cytotoxicity and genotoxicity of the haloacetic acids, a major class of drinking water disinfection by-products[J]. Environmental and Molecular Mutagenesis, 2010, 51(8-9):871-878 Hu S Y, Gong T T, Xian Q M, et al. Formation of iodinated trihalomethanes and haloacetic acids from aromatic iodinated disinfection byproducts during chloramination[J]. Water Research, 2018, 147:254-263 Hu S Y, Gong T T, Wang J J, et al. Trihalomethane yields from twelve aromatic halogenated disinfection byproducts during chlor(am)ination[J]. Chemosphere, 2019, 228:668-675 Pan Y, Li W B, An H, et al. Formation and occurrence of new polar iodinated disinfection byproducts in drinking water[J]. Chemosphere, 2016, 144:2312-2320 Wang W, Qian Y C, Jmaiff L K, et al. Precursors of halobenzoquinones and their removal during drinking water treatment processes[J]. Environmental Science & Technology, 2015, 49(16):9898-9904 Zhao Y L, Anichina J, Lu X F, et al. Occurrence and formation of chloro- and bromo-benzoquinones during drinking water disinfection[J]. Water Research, 2012, 46(14):4351-4360 Zhang D, Chu W H, Yu Y, et al. Occurrence and stability of chlorophenylacetonitriles, a new class of nitrogenous aromatic DBPs, in chlorinated and chloraminated drinking waters[J]. Environmental Science & Technology Letters, 2018, 5(6):394-399 Huang G, Jmaiff L, Jiang P, et al. Formation, identification, and occurrence of new bromo- and mixed halo-tyrosyl dipeptides in chloraminated water[J]. Environmental Science & Technology, 2019, 53(7):3672-3680 Tian D Y, Moe B, Huang G, et al. Cytotoxicity of halogenated tyrosyl compounds, an emerging class of disinfection byproducts[J]. Chemical Research in Toxicology, 2020, 33(4):1028-1035 Wang W, Qian Y C, Li J H, et al. Analytical and toxicity characterization of halo-hydroxyl-benzoquinones as stable halobenzoquinone disinfection byproducts in treated water[J]. Analytical Chemistry, 2014, 86(10):4982-4988 Wang W, Qian Y C, Boyd J M, et al. Halobenzoquinones in swimming pool waters and their formation from personal care products[J]. Environmental Science & Technology, 2013, 47(7):3275-3282 Zhang Z X, Zhu Q Y, Huang C, et al. Comparative cytotoxicity of halogenated aromatic DBPs and implications of the corresponding developed QSAR model to toxicity mechanisms of those DBPs:Binding interactions between aromatic DBPs and catalase play an important role[J]. Water Research, 2020, 170:115283 Hung S, Mohan A, Reckhow D A, et al. Assessment of the in vitro toxicity of the disinfection byproduct 2,6-dichloro-1,4-benzoquinone and its transformed derivatives[J]. Chemosphere, 2019, 234:902-908 Du H Y, Li J H, Moe B, et al. Cytotoxicity and oxidative damage induced by halobenzoquinones to T24 bladder cancer cells[J]. Environmental Science & Technology, 2013, 47(6):2823-2830 Du H Y, Li J H, Moe B, et al. A real-time cell-electronic sensing method for comparative analysis of toxicity of water contaminants[J]. Anal Methods, 2014, 6(7):2053-2058 Li J H, Moe B, Vemula S, et al. Emerging disinfection byproducts, halobenzoquinones:Effects of isomeric structure and halogen substitution on cytotoxicity, formation of reactive oxygen species, and genotoxicity[J]. Environmental Science & Technology, 2016, 50(13):6744-6752 Procházka E, Escher B I, Plewa M J, et al. In vitro cytotoxicity and adaptive stress responses to selected haloacetic acid and halobenzoquinone water disinfection byproducts[J]. Chemical Research in Toxicology, 2015, 28(10):2059-2068 Attene-Ramos M S, Wagner E D, Plewa M J. Comparative human cell toxicogenomic analysis of monohaloacetic acid drinking water disinfection byproducts[J]. Environmental Science & Technology, 2010, 44(19):7206-7212 Vlastos D, Antonopoulou M, Konstantinou I. Evaluation of toxicity and genotoxicity of 2-chlorophenol on bacteria, fish and human cells[J]. Science of the Total Environment, 2016, 551-552:649-655 Chen Y H, Qin L T, Mo L Y, et al. Synergetic effects of novel aromatic brominated and chlorinated disinfection byproducts on Vibrio qinghaiensis sp.-Q67[J]. Environmental Pollution, 2019, 250:375-385 Li X Z, Wang M E, Chen W P, et al. Evaluation of combined toxicity of siduron and cadmium on earthworm (Eisenia fetida) using biomarker response index[J]. Science of the Total Environment, 2019, 646:893-901 Zhang Z X, Yang M T, Yi J Y, et al. Comprehensive insights into the interactions of two emerging bromophenolic DBPs with human serum albumin by multispectroscopy and molecular docking[J]. ACS Omega, 2019, 4(1):563-572 Liberatore H K, Plewa M J, Wagner E D, et al. Identification and comparative mammalian cell cytotoxicity of new iodo-phenolic disinfection byproducts in chloraminated oil and gas wastewaters[J]. Environmental Science & Technology Letters, 2017, 4(11):475-480 Zuo Y T, Hu Y, Lu W W, et al. Toxicity of 2,6-dichloro-1,4-benzoquinone and five regulated drinking water disinfection by-products for the Caenorhabditis elegans nematode[J]. Journal of Hazardous Materials, 2017, 321:456-463 Shehata M, Durner J, Thiessen D, et al. Induction of DNA double-strand breaks by monochlorophenol isomers and ChKM in human gingival fibroblasts[J]. Archives of Toxicology, 2012, 86(9):1423-1429 Yin D Q, Zhu H K, Hu P, et al. Genotoxic effect of 2,4,6-trichlorophenol on p53 gene in zebrafish liver[J]. Environmental Toxicology and Chemistry, 2009, 28(3):603-608 Jansson K, Jansson V. Genotoxicity of 2,4,6-trichlorophenol in V79 Chinese hamster cells[J]. Mutation Research/Genetic Toxicology, 1992, 280(3):175-179 Igbinosa E O, Odjadjare E E, Chigor V N, et al. Toxicological profile of chlorophenols and their derivatives in the environment:The public health perspective[J]. The Scientific World Journal, 2013, 2013:460215 Cooke M S, Evans M D, Dizdaroglu M, et al. Oxidative DNA damage:Mechanisms, mutation, and disease[J]. FASEB Journal, 2003, 17(10):1195-1214 Horn H F, Vousden K H. Coping with stress:Multiple ways to activate p53[J]. Oncogene, 2007, 26(9):1306-1316 Levine A J. p53, the cellular gatekeeper for growth and division[J]. Cell, 1997, 88(3):323-331 Klaunig J E, Kamendulis L M, Hocevar B A. Oxidative stress and oxidative damage in carcinogenesis[J]. Toxicologic Pathology, 2010, 38(1):96-109 Storz P. Reactive oxygen species in tumor progression[J]. Frontiers in Bioscience, 2005, 10:1881-1896 Eruslanov E, Kusmartsev S. Identification of ROS Using Oxidized DCFDA and Flow-cytometry[M]//. Advanced Protocols in Oxidative Stress Ⅱ. Springer, 2010:57-72 Li J H, Wang W, Moe B, et al. Chemical and toxicological characterization of halobenzoquinones, an emerging class of disinfection byproducts[J]. Chemical Research in Toxicology, 2015, 28(3):306-318 Liu J, Song E Q, Liu L C, et al. Polychlorinated biphenyl quinone metabolites lead to oxidative stress in HepG2 cells and the protective role of dihydrolipoic acid[J]. Toxicology in Vitro, 2012, 26(6):841-848 Yang H B, Zhao Y Z, Tang Y, et al. Antioxidant defence system is responsible for the toxicological interactions of mixtures:A case study on PFOS and PFOA in Daphnia magna[J]. Science of the Total Environment, 2019, 667:435-443 Lou J X, Wang W, Zhu L Z. Occurrence, formation, and oxidative stress of emerging disinfection byproducts, halobenzoquinones, in tea[J]. Environmental Science & Technology, 2019, 53(20):11860-11868 Li J H, Wang W, Zhang H Q, et al. Glutathione-mediated detoxification of halobenzoquinone drinking water disinfection byproducts in T24 cells[J]. Toxicological Sciences, 2014, 141(2):335-343 Wang W, Qian Y C, Li J H, et al. Characterization of mechanisms of glutathione conjugation with halobenzoquinones in solution and HepG2 cells[J]. Environmental Science & Technology, 2018, 52(5):2898-2908 Baigi M G, Brault L, Néguesque A, et al. Apoptosis/necrosis switch in two different cancer cell lines:Influence of benzoquinone- and hydrogen peroxide-induced oxidative stress intensity, and glutathione[J]. Toxicology in Vitro, 2008, 22(6):1547-1554 Li J H, Moe B, Liu Y M, et al. Halobenzoquinone-induced alteration of gene expression associated with oxidative stress signaling pathways[J]. Environmental Science & Technology, 2018, 52(11):6576-6584 Kundu B, Richardson S D, Granville C A, et al. Comparative mutagenicity of halomethanes and halonitromethanes in Salmonella TA100:Structure-activity analysis and mutation spectra[J]. Fundamental and Molecular Mechanisms of Mutagenesis, 2004, 554(1-2):335-350 伍吉云, 万祎, 胡建英. 环境中内分泌干扰物的作用机制[J]. 环境与健康杂志, 2005, 22(6):494-497 Wu J Y, Wan Y, Hu J Y. The action mechanism of environmental endocrine disruptors[J]. Journal of Environment and Health, 2005, 22(6):494-497(in Chinese)
Ng H W, Perkins R, Tong W D, et al. Versatility or promiscuity:The estrogen receptors, control of ligand selectivity and an update on subtype selective ligands[J]. International Journal of Environmental Research and Public Health, 2014, 11(9):8709-8742 Hilscherova K, Jones P D, Gracia T, et al. Assessment of the effects of chemicals on the expression of ten steroidogenic genes in the H295R cell line using real-time PCR[J]. Toxicological Sciences, 2004, 81(1):78-89 Zhang X W, Yu R M K, Jones P D, et al. Quantitative RT-PCR methods for evaluating toxicant-induced effects on steroidogenesis using the H295R cell line[J]. Environmental Science & Technology, 2005, 39(8):2777-2785 Ezechiáš M, Svobodová K, Cajthaml T. Hormonal activities of new brominated flame retardants[J]. Chemosphere, 2012, 87(7):820-824 Hamers T, Kamstra J H, Sonneveld E, et al. In vitro profiling of the endocrine-disrupting potency of brominated flame retardants[J]. Toxicological Sciences, 2006, 92(1):157-173 Leusch F D L, Neale P A, Hebert A, et al. Analysis of the sensitivity of in vitro bioassays for androgenic, progestagenic, glucocorticoid, thyroid and estrogenic activity:Suitability for drinking and environmental waters[J]. Environment International, 2017, 99:120-130 Deng J, Liu C S, Yu L Q, et al. Chronic exposure to environmental levels of tribromophenol impairs zebrafish reproduction[J]. Toxicology and Applied Pharmacology, 2010, 243(1):87-95 Olsen C M, Meussen-Elholm E T M, Holme J A, et al. Brominated phenols:Characterization of estrogen-like activity in the human breast cancer cell-line MCF-7[J]. Toxicology Letters, 2002, 129(1-2):55-63 Holmes B E, Smeester L, Fry R C, et al. Identification of endocrine active disinfection by-products (DBPs) that bind to the androgen receptor[J]. Chemosphere, 2017, 187:114-122 Holmes B E, Smeester L, Fry R C, et al. Disinfection byproducts bind human estrogen receptor-α[J]. Environmental Toxicology and Chemistry, 2019, 38(5):956-964 Yang X H, Ou W, Xi Y, et al. Emerging polar phenolic disinfection byproducts are high-affinity human transthyretin disruptors:An in vitro and in silico study[J]. Environmental Science & Technology, 2019, 53(12):7019-7028 Kollitz E M, De Carbonnel L, Stapleton H M, et al. The affinity of brominated phenolic compounds for human and zebrafish thyroid receptor β:Influence of chemical structure[J]. Toxicological Sciences, 2018, 163(1):226-239 Kudo Y, Yamauchi K. In vitro and in vivo analysis of the thyroid disrupting activities of phenolic and phenol compounds in Xenopus laevis[J]. Toxicological Sciences, 2005, 84(1):29-37 Suzuki G, Takigami H, Watanabe M, et al. Identification of brominated and chlorinated phenols as potential thyroid-disrupting compounds in indoor dusts[J]. Environmental Science & Technology, 2008, 42(5):1794-1800 Lee D, Ahn C, Hong E J, et al. 2,4,6-tribromophenol interferes with the thyroid hormone system by regulating thyroid hormones and the responsible genes in mice[J]. International Journal of Environmental Research and Public Health, 2016, 13(7):697 Xi Y, Yang X H, Zhang H Y, et al. Binding interactions of halo-benzoic acids, halo-benzenesulfonic acids and halo-phenylboronic acids with human transthyretin[J]. Chemosphere, 2020, 242:125135 Hassenklöver T, Predehl S, Pilli J, et al. Bromophenols, both present in marine organisms and in industrial flame retardants, disturb cellular Ca2+ signaling in neuroendocrine cells (PC12)[J]. Aquatic Toxicology, 2006, 76(1):37-45 Li N, Jiang W W, Ma M, et al. Chlorination by-products of bisphenol A enhanced retinoid X receptor disrupting effects[J]. Journal of Hazardous Materials, 2016, 320:289-295 Kolšek K, Mavri J, Sollner Dolenc M, et al. Endocrine disruptome-An open source prediction tool for assessing endocrine disruption potential through nuclear receptor binding[J]. Journal of Chemical Information and Modeling, 2014, 54(4):1254-1267 Chen Q C, Tan H Y, Yu H X, et al. Activation of steroid hormone receptors:Shed light on the in silico evaluation of endocrine disrupting chemicals[J]. Science of the Total Environment, 2018, 631-632:27-39 Li Y, Jiang J Y, Li W X, et al. Volatile DBPs contributed marginally to the developmental toxicity of drinking water DBP mixtures against Platynereis dumerilii[J]. Chemosphere, 2020, 252:126611 Liu J Q, Zhang X R, Li Y. Effect of Boiling on Halogenated DBPs and Their Developmental Toxicity in Real Tap Waters[M]//ACS Symposium Series. Washington DC:American Chemical Society, 2015:45-60 Pan Y, Zhang X R, Wagner E D, et al. Boiling of simulated tap water:Effect on polar brominated disinfection byproducts, halogen speciation, and cytotoxicity[J]. Environmental Science & Technology, 2014, 48(1):149-156 Yang M T, Zhang X R. Comparative developmental toxicity of new aromatic halogenated DBPs in a chlorinated saline sewage effluent to the marine polychaete Platynereis dumerilii[J]. Environmental Science & Technology, 2013, 47(19):10868-10876 Pan Y, Zhang X R, Li Y. Identification, toxicity and control of iodinated disinfection byproducts in cooking with simulated chlor(am)inated tap water and iodized table salt[J]. Water Research, 2016, 88:60-68 Wang C, Yang X, Zheng Q, et al. Halobenzoquinone-induced developmental toxicity, oxidative stress, and apoptosis in zebrafish embryos[J]. Environmental Science & Technology, 2018, 52(18):10590-10598 Liu J Q, Zhang X R. Comparative toxicity of new halophenolic DBPs in chlorinated saline wastewater effluents against a marine alga:Halophenolic DBPs are generally more toxic than haloaliphatic ones[J]. Water Research, 2014, 65:64-72 Xie Y C, Jiang L J, Qiu J F, et al. A comparative evaluation of the immunotoxicity and immunomodulatory effects on macrophages exposed to aromatic trihalogenated DBPs[J]. Immunopharmacology and Immunotoxicology, 2019, 41(2):319-326 Bull R J, Reckhow D A, Li X F, et al. Potential carcinogenic hazards of non-regulated disinfection by-products:Haloquinones, halo-cyclopentene and cyclohexene derivatives, N-halamines, halonitriles, and heterocyclic amines[J]. Toxicology, 2011, 286(1-3):1-19 Chen B Y, Zhang T, Bond T, et al. Development of quantitative structure activity relationship (QSAR) model for disinfection byproduct (DBP) research:A review of methods and resources[J]. Journal of Hazardous Materials, 2015, 299:260-279 Tuppurainen K, Lötjönen S, Laatikainen R, et al. About the mutagenicity of chlorine-substituted furanones and halopropenals. A QSAR study using molecular orbital indices[J]. Fundamental and Molecular Mechanisms of Mutagenesis, 1991, 247(1):97-102 Méndez-Hernández D D, Tarakeshwar P, Gust D, et al. Simple and accurate correlation of experimental redox potentials and DFT-calculated HOMO/LUMO energies of polycyclic aromatic hydrocarbons[J]. Journal of Molecular Modeling, 2013, 19(7):2845-2848 Chan K T, Jensen N S, Silber P M, et al. Structure-activity relationships for halobenzene induced cytotoxicity in rat and human hepatoctyes[J]. Chemico-Biological Interactions, 2007, 165(3):165-174 -
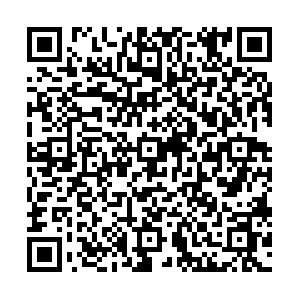
计量
- 文章访问数: 3893
- HTML全文浏览数: 3893
- PDF下载数: 97
- 施引文献: 0