环境微生物耐药阻控研究进展
Progress on Antibiotic Resistance Control of Environmental Microorganisms
-
摘要: 世界卫生组织将微生物耐药视为21世纪全球健康的主要挑战之一。根据One Health观念,微生物耐药可以跨越生态边界在环境、动物、人体中传播,给生态安全和人类健康带来威胁。耐药菌株的快速传播和演变使得传统抗生素治疗效果逐渐减弱。因此,需要研究环境中耐药现状,提出缓解环境微生物耐药的策略。在该综述中,我们重点介绍缓解微生物耐药的策略,具体包括化学阻控、生物阻控、CRISPR-Cas系统应用和群落调节等手段,并对这些策略的优缺点进行了评述。这些策略有望遏制全球耐药的恶化及蔓延。Abstract: Antibiotic resistance has been identified by the World Health Organization as one of the major global health challenges of the 21st Century. From a One Health perspective, the spread of antimicrobial resistance across ecological boundaries poses significant threats to human health and ecosystems. The efficacy of traditional antibiotics has been diminished by the rapid dissemination and evolution of resistant strains. Therefore, it is imperative that the current status of environmental antibiotic resistance be assessed and strategies to mitigate it be developed. In this review, several strategies to address antimicrobial resistance are examined, including chemical resistance control, biological resistance control, CRISPR-Cas system application, and community regulation. The advantages and disadvantages of each approach are evaluated. It is expected that these strategies will effectively curb the deterioration and spread of global drug resistance.
-
-
Darby E M, Trampari E, Siasat P, et al. Molecular mechanisms of antibiotic resistance revisited[J]. Nature Reviews Microbiology, 2023, 21(5): 280-295 Antimicrobial Resistance Collaborators. Global burden of bacterial antimicrobial resistance in 2019: A systematic analysis[J]. Lancet, 2022, 399(10325): 629-655 Robinson T P, Bu D P, Carrique-Mas J, et al. Antibiotic resistance is the quintessential One Health issue[J]. Transactions of the Royal Society of Tropical Medicine and Hygiene, 2016, 110(7): 377-380 Wernli D, Jørgensen P S, Morel C M, et al. Mapping global policy discourse on antimicrobial resistance[J]. BMJ Global Health, 2017, 2(2): e000378 Kapoor G, Saigal S, Elongavan A. Action and resistance mechanisms of antibiotics: A guide for clinicians[J]. Journal of Anaesthesiology, Clinical Pharmacology, 2017, 33(3): 300-305 Liao P, Zhan Z Y, Dai J, et al. Adsorption of tetracycline and chloramphenicol in aqueous solutions by bamboo charcoal: A batch and fixed-bed column study[J]. Chemical Engineering Journal, 2013, 228: 496-505 王芳, 豆庆圆, 付玉豪, 等. 土壤中有机肥源抗生素抗性基因环境归趋与风险管理研究进展[J]. 农业环境科学学报, 2022, 41(12): 2563-2576 Wang F, Dou Q Y, Fu Y H, et al. Environmental fate and risk management of manure-borne antibiotic resistance genes in soil: A review[J]. Journal of Agro-Environment Science, 2022, 41(12): 2563-2576(in Chinese)
Martínez J L. Antibiotics and antibiotic resistance genes in natural environments[J]. Science, 2008, 321(5887): 365-367 Tang K L, Caffrey N P, Nóbrega D B, et al. Restricting the use of antibiotics in food-producing animals and its associations with antibiotic resistance in food-producing animals and human beings: A systematic review and meta-analysis[J]. The Lancet Planetary Health, 2017, 1(8): e316-e327 Enne V I, Livermore D M, Stephens P, et al. Persistence of sulphonamide resistance in Escherichia coli in the UK despite national prescribing restriction[J]. Lancet, 2001, 357(9265): 1325-1328 Hernando-Amado S, Coque T M, Baquero F, et al. Defining and combating antibiotic resistance from One Health and Global Health perspectives[J]. Nature Microbiology, 2019, 4(9): 1432-1442 Ali N, Lin Y F, Jiang L G, et al. Biochar and manure applications differentially altered the class 1 integrons, antimicrobial resistance, and gene cassettes diversity in paddy soils[J]. Frontiers in Microbiology, 2022, 13: 943880 Yaashikaa P R, Kumar P S, Varjani S, et al. A critical review on the biochar production techniques, characterization, stability and applications for circular bioeconomy[J]. Biotechnology Reports (Amsterdam, Netherlands), 2020, 28: e00570 Guo X X, Liu H T, Zhang J. The role of biochar in organic waste composting and soil improvement: A review[J]. Waste Management, 2020, 102: 884-899 Sun F L, Xu Z T, Fan L L. Response of heavy metal and antibiotic resistance genes and related microorganisms to different heavy metals in activated sludge[J]. Journal of Environmental Management, 2021, 300: 113754 He X Q, Xiong J P, Yang Z L, et al. Exploring the impact of biochar on antibiotics and antibiotics resistance genes in pig manure aerobic composting through untargeted metabolomics and metagenomics[J]. Bioresource Technology, 2022, 352: 127118 Awasthi M K, Liu H, Liu T, et al. Effect of biochar addition on the dynamics of antibiotic resistant bacteria during the pig manure composting[J]. Science of the Total Environment, 2022, 814: 152688 Wang C H, Wang Y F, Yan S, et al. Biochar-amended composting of lincomycin fermentation dregs promoted microbial metabolism and reduced antibiotic resistance genes[J]. Bioresource Technology, 2023, 367: 128253 Duan M L, Li H C, Gu J, et al. Effects of biochar on reducing the abundance of oxytetracycline, antibiotic resistance genes, and human pathogenic bacteria in soil and lettuce[J]. Environmental Pollution, 2017, 224: 787-795 Wu C Y, Fu L Y, Li H Q, et al. Using biochar to strengthen the removal of antibiotic resistance genes: Performance and mechanism[J]. Science of the Total Environment, 2022, 816: 151554 Wu Y J, Yan H C, Zhu X M, et al. Biochar effectively inhibits the horizontal transfer of antibiotic resistance genes via restraining the energy supply for conjugative plasmid transfer[J]. Environmental Science & Technology, 2022, 56(17): 12573-12583 Liang J L, Shan C, Zhang X, et al. Bactericidal mechanism of BiOI-AgI under visible light irradiation[J]. Chemical Engineering Journal, 2015, 279: 277-285 Xie M M, Gao M, Yun Y, et al. Antibacterial nanomaterials: Mechanisms, impacts on antimicrobial resistance and design principles[J]. Angewandte Chemie (International Ed in English), 2023, 62(17): e202217345 Garland M, Loscher S, Bogyo M. Chemical strategies to target bacterial virulence[J]. Chemical Reviews, 2017, 117(5): 4422-4461 He J, Hong M, Xie W Q, et al. Progress and prospects of nanomaterials against resistant bacteria[J]. Journal of Controlled Release, 2022, 351: 301-323 Nederberg F, Zhang Y, Tan J P, et al. Biodegradable nanostructures with selectivelysis of microbial membranes[J]. Nature Chemistry, 2011, 3(5): 409-414 Zhao H R, Wang M L, Cui Y T, et al. Can we arrest the evolution of antibiotic resistance? The differences between the effects of silver nanoparticles and silver ions[J]. Environmental Science & Technology, 2022, 56(8): 5090-5101 Kümmerer K, Menz J, Schubert T, et al. Biodegradability of organic nanoparticles in the aqueous environment[J]. Chemosphere, 2011, 82(10): 1387-1392 Zhou Z R, Lian Y L, Zhu L, et al. Platinum nanoparticles prevent the resistance of Pseudomonas aeruginosa to ciprofloxacin and imipenem: Mechanism insights[J]. ACS Nano, 2023, 17(24): 24685-24695 Chen P P, Yu X F, Zhang J Y, et al. New and traditional methods for antibiotic resistance genes removal: Constructed wetland technology and photocatalysis technology[J]. Frontiers in Microbiology, 2023, 13: 1110793 Moreira N F F, Narciso-da-Rocha C, Polo-López M I, et al. Solar treatment (H2O2, TiO2-P25 and GO-TiO2 photocatalysis, photo-Fenton) of organic micropollutants, human pathogen indicators, antibiotic resistant bacteria and related genes in urban wastewater[J]. Water Research, 2018, 135: 195-206 Guo M T, Tian X B. Impacts on antibiotic-resistant bacteria and their horizontal gene transfer by graphene-based TiO2&Ag composite photocatalysts under solar irradiation[J]. Journal of Hazardous Materials, 2019, 380: 120877 Zhou Z R, Shen Z R, Cheng Z H, et al. Mechanistic insights for efficient inactivation of antibiotic resistance genes: A synergistic interfacial adsorption and photocatalytic-oxidation process[J]. Science Bulletin, 2020, 65(24): 2107-2119 Ren S J, Boo C, Guo N, et al. Photocatalytic reactive ultrafiltration membrane for removal of antibiotic resistant bacteria and antibiotic resistance genes from wastewater effluent[J]. Environmental Science & Technology, 2018, 52(15): 8666-8673 Ji H, Cai Y W, Wang Z X, et al. Sub-lethal photocatalysis promotes horizontal transfer of antibiotic resistance genes by conjugation and transformability[J]. Water Research, 2022, 221: 118808 Garvey N, St John A C, Witkin E M. Evidence for RecA protein association with the cell membrane and for changes in the levels of major outer membrane proteins in SOS-induced Escherichia coli cells[J]. Journal of Bacteriology, 1985, 163(3): 870-876 Yin H L, Chen X F, Li G Y, et al. Can photocatalytic technology facilitate conjugative transfer of ARGs in bacteria at the interface of natural sphalerite under different light irradiation?[J]. Applied Catalysis B: Environmental, 2021, 287: 119977 Shatzkes K, Singleton E, Tang C, et al. Predatory bacteria attenuate Klebsiella pneumoniae burden in rat lungs[J]. mBio, 2016, 7(6): e01847-16 Atterbury R J, Tyson J. Predatory bacteria as living antibiotics: Where are we now?[J]. Microbiology (Reading, England), 2021, 167(1): 1 Lambina V A, Afinogenova A V, Romaǐ Penabad S, et al. Micavibrio admirandus gen. et sp. nov[J]. Mikrobiologiia, 1982, 51(1): 114-117 Thiery S, Kaimer C. The predation strategy of Myxococcus xanthus[J]. Frontiers in Microbiology, 2020, 11: 2 Arend K I, Schmidt J J, Bentler T, et al. Myxococcus xanthus predation of Gram-positive or Gram-negative bacteria is mediated by different bacteriolytic mechanisms[J]. Applied and Environmental Microbiology, 2021, 87(5): e02382-20 Thiery S, Turowski P, Berleman J E, et al. The predatory soil bacterium Myxococcus xanthus combines a Tad- and an atypical type 3-like protein secretion system to kill bacterial cells[J]. Cell Reports, 2022, 40(11): 111340 Shanks R M, Davra V R, Romanowski E G, et al. An eye to a kill: Using predatory bacteria to control Gram-negative pathogens associated with ocular infections[J]. PLoS One, 2013, 8(6): e66723 Dwidar M, Monnappa A K, Mitchell R J. The dual probiotic and antibiotic nature of Bdellovibrio bacteriovorus[J]. BMB Reports, 2012, 45(2): 71-78 Saralegui C, Herencias C, Halperin A V, et al. Strain-specific predation of Bdellovibrio bacteriovorus on Pseudomonas aeruginosa with a higher range for cystic fibrosis than for bacteremia isolates[J]. Scientific Reports, 2022, 12(1): 10523 Pérez-Acevedo G, Bosch-Alcaraz A, Torra-Bou J E. Larval therapy for treatment of chronic wounds colonized by multi-resistant pathogens in a pediatric patient: A case study[J]. Journal of Wound, Ostomy, and Continence Nursing, 2022, 49(4): 373-378 Negus D, Moore C, Baker M, et al. Predator versus pathogen: How does predatory Bdellovibrio bacteriovorus interface with the challenges of killing Gram-negative pathogens in a host setting?[J]. Annual Review of Microbiology, 2017, 71: 441-457 Keen E C. A century of phage research: Bacteriophages and the shaping of modern biology[J]. BioEssays, 2015, 37(1): 6-9 Pratama A A, van Elsas J D. The 'neglected’ soil virome-potential role and impact[J]. Trends in Microbiology, 2018, 26(8): 649-662 MacNair C R, Rutherford S T, Tan M W. Alternative therapeutic strategies to treat antibiotic-resistant pathogens[J]. Nature Reviews Microbiology, 2024, 22(5): 262-275 Dedrick R M, Guerrero-Bustamante C A, Garlena R A, et al. Engineered bacteriophages for treatment of a patient with a disseminated drug-resistant Mycobacterium abscessus[J]. Nature Medicine, 2019, 25(5): 730-733 Nick J A, Dedrick R M, Gray A L, et al. Host and pathogen response to bacteriophage engineered against Mycobacterium abscessus lung infection[J]. Cell, 2022, 185(11): 1860-1874.e12 Jault P, Leclerc T, Jennes S, et al. Efficacy and tolerability of a cocktail of bacteriophages to treat burn wounds infected by Pseudomonas aeruginosa (PhagoBurn): A randomised, controlled, double-blind phase 1/2 trial[J]. The Lancet Infectious Diseases, 2019, 19(1): 35-45 Leitner L, Ujmajuridze A, Chanishvili N, et al. Intravesical bacteriophages for treating urinary tract infections in patients undergoing transurethral resection of the prostate: A randomised, placebo-controlled, double-blind clinical trial[J]. The Lancet Infectious Diseases, 2021, 21(3): 427-436 Rodríguez-Rubio L, Jofre J, Muniesa M. Is genetic mobilization considered when using bacteriophages in antimicrobial therapy?[J]. Antibiotics (Basel, Switzerland), 2017, 6(4): 32 Morris T C, Reyneke B, Havenga B, et al. Simultaneous Bdellovibrio bacteriovorus: Bacteriophage dosing with SODIS for treatment of environmental water sources[J]. Journal of Environmental Chemical Engineering, 2024, 12(2): 112359 Hobley L, Summers J K, Till R, et al. Dual predation by bacteriophage and Bdellovibrio bacteriovorus can eradicate Escherichia coli prey in situ ations where single predation cannot[J]. Journal of Bacteriology, 2020, 202(6): e00629-19 Shuai X Y, Zhou Z C, Ba X L, et al. Bacteriophages: Vectors of or weapons against the transmission of antibiotic resistance genes in hospital wastewater systems?[J]. Water Research, 2024, 248: 120833 Marchfelder A. Special focus CRISPR-cas[J]. RNA Biology, 2013, 10(5): 655-658 Wu Y Y, Battalapalli D, Hakeem M J, et al. Engineered CRISPR-Cas systems for the detection and control of antibiotic-resistant infections[J]. Journal of Nanobiotechnology, 2021, 19(1): 401 Hao M J, He Y Z, Zhang H F, et al. CRISPR-Cas9-mediated carbapenemase gene and plasmid curing in carbapenem-resistantEnterobacteriaceae[J]. Antimicrobial Agents and Chemotherapy, 2020, 64(9): e00843-20 He Y Z, Kuang X, Long T F, et al. Re-engineering a mobile-CRISPR/Cas9 system for antimicrobial resistance gene curing and immunization in Escherichia coli[J]. Journal of Antimicrobial Chemotherapy, 2021, 77(1): 74-82 Li P S, Wan P, Zhao R N, et al. Targeted elimination of blaNDM-5 gene in Escherichia coli by conjugative CRISPR-Cas9 system[J]. Infection and Drug Resistance, 2022, 15: 1707-1716 Almendros C, Nobrega F L, McKenzie R E, et al. Cas4-Cas1 fusions drive efficient PAM selection and control CRISPR adaptation[J]. Nucleic Acids Research, 2019, 47(10): 5223-5230 Park J Y, Moon B Y, Park J W, et al. Genetic engineering of a temperate phage-based delivery system for CRISPR/Cas9 antimicrobials against Staphylococcus aureus[J]. Scientific Reports, 2017, 7: 44929 Gomaa A A, Klumpe H E, Luo M L, et al. Programmable removal of bacterial strains by use of genome-targeting CRISPR-Cas systems[J]. mBio, 2014, 5(1): e00928-13 李虎良, 张蕾. 抗生素耐药性的分子机制及抑菌策略[J]. 中国生物化学与分子生物学报, 2024, 40(6): 759-769 Li H L, Zhang L. Molecular mechanism and antimicrobial strategy of antibiotic resistance[J]. Chinese Journal of Biochemistry and Molecular Biology, 2024, 40(6): 579-769(in Chinese)
Esparza I, Jiménez-Moreno N, Bimbela F, et al. Fruit and vegetable waste management: Conventional and emerging approaches[J]. Journal of Environmental Management, 2020, 265: 110510 De Corato U. Agricultural waste recycling in horticultural intensive farming systems by on-farm composting and compost-based tea application improves soil quality and plant health: A review under the perspective of a circular economy[J]. Science of the Total Environment, 2020, 738: 139840 Lin D, Huang D, Zhang J H, et al. Reduction of antibiotic resistance genes (ARGs) in swine manure-fertilized soil via fermentation broth from fruit and vegetable waste[J]. Environmental Research, 2022, 214(Pt 1): 113835 Lin D, Zhu L, Yao Y L, et al. The ecological and molecular mechanism underlying effective reduction of antibiotic resistance genes pollution in soil by fermentation broth from fruit and vegetable waste[J]. Journal of Hazardous Materials, 2023, 451: 131201 -
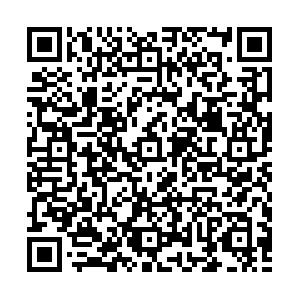
计量
- 文章访问数: 987
- HTML全文浏览数: 987
- PDF下载数: 183
- 施引文献: 0