有机肥施用土壤-蔬菜系统中潜在人畜病原菌及其耐药性分析
Analysis of Potential Human and Zoonotic Pathogens and Their Resistance to Antibiotics in Organic Fertilizer Applied Soil-Vegetable System
-
摘要: 畜禽粪便类有机肥中含有大量的人畜病原菌和抗生素抗性基因(antibiotic resistance genes, ARGs),施入农田后部分病原菌仍能继续存活并繁殖,且可能污染所种植的蔬菜,尤其是一些具有耐药性的人畜病原菌经食物链传递后将对人体健康造成巨大威胁。然而,当前大量的研究仅基于单纯的分离培养或高通量测序技术,对有机肥施用土壤-蔬菜系统中的人畜病原菌及其携带的毒力因子基因(virulence factor genes, VFGs)与ARGs认识有限。因此,本研究针对典型的长期施用有机肥的土壤-蔬菜系统,采集有机肥、施肥土及该土壤上种植的叶类蔬菜,如鸡毛菜、菜心、油麦菜和白菜等,结合传统分离培养及全基因组测序(whole genome sequencing, WGS)技术,利用生物信息学方法对分离菌株进行分类学及基因组注释,探究人畜病原菌的分布以及VFGs和ARGs的携带情况。结果表明在该施用有机肥的土壤-蔬菜系统中分离检测到42株人畜病原菌,其中37株属于肠杆菌科,5株属于假单孢菌科,主要的菌株包括大肠杆菌、克雷伯氏菌、假单胞菌和柠檬酸杆菌等,其中,大肠杆菌在土壤环境中显著富集,假单胞菌和柠檬酸杆菌主要分布在蔬菜根际及叶际。此外,全基因组测序提供了病原菌遗传信息,表明了人畜病原菌可能具有潜在的攻击性和代谢活性,携带有效应子、粘附性、生物膜、免疫调节性、侵袭性及毒素相关的VFGs。更重要的是,这些人畜病原菌均携带多种ARGs,这些ARGs编码了对多重耐药类、β-内酰胺类、氨基糖苷类、磷霉素类和多黏菌素类等抗生素的抗性。综上,本研究揭示了有机肥施用导致的土壤-蔬菜系统中人畜病原菌的分布情况,详细解析了病原菌携带的VFGs与ARGs类型,指明了食用叶类蔬菜存在的潜在健康风险,为加强畜禽粪便有机肥中重要人畜病原菌的风险防控提供了理论依据。Abstract: Livestock manure-based organic fertilizers are known to harbor a variety of zoonotic pathogens and antibiotic resistance genes (ARGs). When applied to agricultural fields, some of these pathogens can persist, multiply, and potentially contaminate the cultivated vegetables. Notably, the transmission of antibiotic-resistant zoonotic pathogens through the food chain poses a substantial threat to human health. However, the current research predominantly relying on isolation culture or high-throughput sequencing techniques, it provides limited insights into the zoonotic pathogens and the virulence factor genes (VFGs) and ARGs they carry within the soil-vegetable system. Therefore, this study focused on a typical long-term soil-vegetable system applied with organic fertilizer. Organic fertilizer, fertilized soil, and leafy vegetables such as Brassica rapa subsp. pekinensis, Brassica rapa var. parachinensis, Lactuca sativa var. longifoliaf, and Brassica oleracea var. capitata planted in this soil were collected. By combining traditional isolation and cultivation methods with advanced whole genome sequencing (WGS) technology, we classified and annotated the genomes of the isolated strains to explore the prevalence of zoonotic pathogens and the presence of VFGs and ARGs. The results showed that 42 strains of zoonotic pathogens were isolated and detected in the soil-vegetable system applied with organic fertilizer, including Escherichia coli, Klebsiella spp., Pseudomonas spp., and Citrobacter spp. Notably, E. coli was found to be significantly enriched in the soil environment, while Pseudomonas spp. and Citrobacter spp. were predominantly distributed in the rhizosphere and phyllosphere of the vegetables. Additionally, WGS offers comprehensive genetic data of an organism, including genes that suggest high pathogenicity and metabolic activity in zoonotic pathogens. These pathogens carry VFGs associated with effectors, adhesion, biofilm, immune modulation, invasion, and toxin production. More importantly, these zoonotic pathogens also harbor multiple ARGs encoding resistance to diverse antibiotic classes, including multi-drug resistant, β-lactams, aminoglycosides, fosfomycin, and polymyxins. In summary, this study has unveiled the distribution of zoonotic pathogens within the soil-vegetable system after the application of organic fertilizer. It presents a detailed analysis of the VFGs and ARGs carried by these pathogens. The research has also highlighted the potential health risks linked to the consumption of leafy vegetables, providing a theoretical foundation for enhancing risk management and control measures against significant zoonotic pathogens in organic fertilizers derived from livestock manure.
-
-
谢文凤, 吴彤, 石岳骄, 等. 国内外有机肥标准对比及风险评价[J]. 中国生态农业学报(中英文), 2020, 28(12): 1958-1968 Xie W F, Wu T, Shi Y J, et al. Chinese and international organic fertilizer standard comparison and risk assessment[J]. Chinese Journal of Eco-Agriculture, 2020, 28(12): 1958-1968(in Chinese) Black Z, Balta I, Black L, et al. The fate of foodborne pathogens in manure treated soil[J]. Frontiers in Microbiology, 2021, 12: 781357 Brooks J P, Adeli A, McLaughlin M R. Microbial ecology, bacterial pathogens, and antibiotic resistant genes in swine manure wastewater as influenced by three swine management systems[J]. Water Research, 2014, 57: 96-103 李霞, 邓立刚, 王峰恩, 等. 堆肥消减畜禽粪便中病原微生物及抗生素残留的研究进展[J]. 山东农业科学, 2017, 49(7): 161-166 Li X, Deng L G, Wang F E, et al. Progress in removal effect of composting on residues of pathogenic microorganisms and antibiotics in livestock and poultry manure[J]. Shandong Agricultural Sciences, 2017, 49(7): 161-166(in Chinese)
Qian X, Gu J, Sun W, et al. Diversity, abundance, and persistence of antibiotic resistance genes in various types of animal manure following industrial composting[J]. Journal of Hazardous Materials, 2018, 344: 716-722 冯亚辉, 郭姣, 李伊光, 等. 集约化养猪粪便理化性质和堆肥工艺的研究[J]. 中国农业文摘-农业工程, 2023, 35(3): 53-58 Feng Y H, Guo J, Li Y G, et al. Study on the physicochemical properties and composting process of intensive pig manure[J]. Chinese Agricultural Abstracts-Agricultural Engineering, 2023, 35(3), 53-58(in Chinese)
Beuchat L R. Vectors and conditions for preharvest contamination of fruits and vegetables with pathogens capable of causing enteric diseases[J]. British Food Journal, 2006, 108(1): 38-53 Guo Y J, Qiu T L, Gao M, et al. Diversity and abundance of antibiotic resistance genes in rhizosphere soil and endophytes of leafy vegetables: Focusing on the effect of the vegetable species[J]. Journal of Hazardous Materials, 2021, 415: 125595 van Overbeek L, Duhamel M, Aanstoot S, et al. Transmission of Escherichia coli from manure to root zones of field-grown lettuce and leek plants[J]. Microorganisms, 2021, 9(11): 2289 Yang L, Liu W X, Zhu D, et al. Application of biosolids drives the diversity of antibiotic resistance genes in soil and lettuce at harvest[J]. Soil Biology and Biochemistry, 2018, 122: 131-140 Scanes C G. Animals and Human Disease: Zoonosis, Vectors, Food-Borne Diseases, and Allergies[M]//Animals and Human Society. Amsterdam: Elsevier, 2018: 331-354 Liu B, Zheng D D, Jin Q, et al. VFDB 2019: A comparative pathogenomic platform with an interactive web interface[J]. Nucleic Acids Research, 2019, 47(D1): D687-D692 Engering A, Hogerwerf L, Slingenbergh J. Pathogen-host-environment interplay and disease emergence[J]. Emerging Microbes & Infections, 2013, 2(2): e5 Zou Y N, Xiao Z J, Wang L F, et al. Prevalence of antibiotic resistance genes and virulence factors in the sediment of WWTP effluent-dominated rivers[J]. Science of the Total Environment, 2023, 897: 165441 Forsberg K J, Reyes A, Wang B, et al. The shared antibiotic resistome of soil bacteria and human pathogens[J]. Science, 2012, 337(6098): 1107-1111 CDC. What exactly is antibiotic resistance?[EB/OL]. (2022-10-05)[2024-03-05]. https://www.cdc.gov/drugresistance/about.htm 苑学霞, 梁京芸, 范丽霞, 等. 粪肥施用土壤抗生素抗性基因来源、转移及影响因素[J]. 土壤学报, 2020, 57(1): 36-47 Yuan X X, Liang J Y, Fan L X, et al. Effects of manure application on source and transport of antibiotic resistant genes in soil and their affecting factors[J]. Acta Pedologica Sinica, 2020, 57(1): 36-47(in Chinese)
Jechalke S, Schierstaedt J, Becker M, et al. Salmonella establishment in agricultural soil and colonization of crop plants depend on soil type and plant species[J]. Frontiers in Microbiology, 2019, 10: 967 The Huttenhower Lab. KneadData[DB/OL].[2024-03-06]. https://huttenhower.sph.harvard.edu/kneaddata/ Bankevich A, Nurk S, Antipov D, et al. SPAdes: A new genome assembly algorithm and its applications to single-cell sequencing[J]. Journal of Computational Biology, 2012, 19(5): 455-477 Chaumeil P A, Mussig A J, Hugenholtz P, et al. GTDB-Tk v2: Memory friendly classification with the genome taxonomy database[J]. Bioinformatics, 2022, 38(23): 5315-5316 Liu B, Zheng D D, Zhou S Y, et al. VFDB 2022: A general classification scheme for bacterial virulence factors[J]. Nucleic Acids Research, 2022, 50(D1): D912-D917 Yin X L, Zheng X W, Li L G, et al. ARGs-OAP v3.0: Antibiotic-resistance gene database curation and analysis pipeline optimization[J]. Engineering, 2023, 27: 234-241 Zhu L, Lian Y L, Lin D, et al. Insights into microbial contamination in multi-type manure-amended soils: The profile of human bacterial pathogens, virulence factor genes and antibiotic resistance genes[J]. Journal of Hazardous Materials, 2022, 437: 129356 Park S H, Chang P S, Ryu S, et al. Development of a novel selective and differential medium for the isolation of Listeria monocytogenes[J]. Applied and Environmental Microbiology, 2014, 80(3): 1020-1025 余萌, 王似锦, 曹蕊, 等. 洋葱伯克霍尔德菌群(Bcc)的选择和分离培养基研究[J]. 中国药事, 2022, 36(7): 746-757 Yu M, Wang S J, Cao R, et al. Study on selection and subculture agar of Burkholderia cepacia complex[J]. Chinese Pharmaceutical Affairs, 2022, 36(7): 746-757(in Chinese)
Wu J, Guo S M, Li K J, et al. Effect of fertilizer type on antibiotic resistance genes by reshaping the bacterial community and soil properties[J]. Chemosphere, 2023, 336: 139272 Lynch J P 3rd, Clark N M, Zhanel G G. Escalating antimicrobial resistance among Enterobacteriaceae: Focus on carbapenemases[J]. Expert Opinion on Pharmacotherapy, 2021, 22(11): 1455-1473 Al-Kharousi Z S, Guizani N, Al-Sadi A M, et al. Antibiotic resistance of Enterobacteriaceae isolated from fresh fruits and vegetables and characterization of their AmpC β-lactamases[J]. Journal of Food Protection, 2019, 82(11): 1857-1863 Xedzro C, Shimamoto T, Yu L S, et al. Emergence of colistin-resistant Enterobacter cloacae and Raoultella ornithinolytica carrying the phosphoethanolamine transferase gene, mcr-9, derived from vegetables in Japan[J]. Microbiology Spectrum, 2023, 11(6): e0106323 Mancuso G, Midiri A, Gerace E, et al. Bacterial antibiotic resistance: The most critical pathogens[J]. Pathogens, 2021, 10(10): 1310 Ambreetha S, Marimuthu P, Mathee K, et al. Rhizospheric and endophytic Pseudomonas aeruginosa in edible vegetable plants share molecular and metabolic traits with clinical isolates[J]. Journal of Applied Microbiology, 2022, 132(4): 3226-3248 Zhang Y J, Hu H W, Chen Q L, et al. Transfer of antibiotic resistance from manure-amended soils to vegetable microbiomes[J]. Environment International, 2019, 130: 104912 Laborda P, Hernando-Amado S, Martínez J L, et al. Antibiotic Resistance in Pseudomonas[M]//Filloux A, Ramos J L. Pseudomonas aeruginosa: Biology, Pathogenesis and Control Strategies. Cham: Springer International Publishing, 2022: 117-143 Solano C, Echeverz M, Lasa I. Biofilm dispersion and quorum sensing[J]. Current Opinion in Microbiology, 2014, 18: 96-104 Beceiro A, Tomás M, Bou G. Antimicrobial resistance and virulence: A successful or deleterious association in the bacterial world?[J]. Clinical Microbiology Reviews, 2013, 26(2): 185-230 CDC. The biggest antibiotic-resistant threats in the U.S.[EB/OL]. (2022-07-15)[2024-03-12]. https://www.cdc.gov/drugresistance/biggest-threats.html Zhao Y, Su J Q, An X L, et al. Feed additives shift gut microbiota and enrich antibiotic resistance in swine gut[J]. Science of the Total Environment, 2018, 621: 1224-1232 Lima T, Domingues S, Da Silva G J. Manure as a potential hotspot for antibiotic resistance dissemination by horizontal gene transfer events[J]. Veterinary Sciences, 2020, 7(3): 110 金淮, 常志州, 朱述钧. 畜禽粪便中人畜共患病原菌传播的公众健康风险[J]. 江苏农业科学, 2005, 33(3): 103-105 Jin H, Chang Z Z, Zhu S J. Public health risk of transmission of zoonotic pathogens in livestock manure[J]. Jiangsu Agricultural Sciences, 2005, 33(3): 103-105(in Chinese)
Sheng H J, Wang F, Xiang L L, et al. Environmental behavior and control of antibiotic resistance genes in soil[J]. Acta Petrologica Sinica, 2022, 60(1): 39-49 Zhu Y G, Johnson T A, Su J Q, et al. Diverse and abundant antibiotic resistance genes in Chinese swine farms[J]. Proceedings of the National Academy of Sciences of the United States of America, 2013, 110(9): 3435-3440 Liu W B, Ling N, Guo J J, et al. Dynamics of the antibiotic resistome in agricultural soils amended with different sources of animal manures over three consecutive years[J]. Journal of Hazardous Materials, 2021, 401: 123399 Pérez-Valera E, Kyselková M, Ahmed E, et al. Native soil microorganisms hinder the soil enrichment with antibiotic resistance genes following manure applications[J]. Scientific Reports, 2019, 9(1): 6760 Xiao R H, Huang D L, Du L, et al. Antibiotic resistance in soil-plant systems: A review of the source, dissemination, influence factors, and potential exposure risks[J]. Science of the Total Environment, 2023, 869: 161855 Iwu C D, Okoh A I. Preharvest transmission routes of fresh produce associated bacterial pathogens with outbreak potentials: A review[J]. International Journal of Environmental Research and Public Health, 2019, 16(22): 4407 程兆康, 杨金山, 吕敏, 等. 我国畜禽养殖业抗生素的使用特征及其环境与健康风险[J]. 农业资源与环境学报, 2022, 39(6): 1253-1262 Binh C T, Heuer H, Kaupenjohann M, et al. Diverse aadA gene cassettes on class 1 integrons introduced into soil via spread manure[J]. Research in Microbiology, 2009, 160(6): 427-433 Chen Q L, An X L, Zhu Y G, et al. Application of struvite alters the antibiotic resistome in soil, rhizosphere, and phyllosphere[J]. Environmental Science & Technology, 2017, 51(14): 8149-8157 Guo Y J, Qiu T L, Gao M, et al. Diversity and abundance of antibiotic resistance genes in rhizosphere soil and endophytes of leafy vegetables: Focusing on the effect of the vegetable species[J]. Journal of Hazardous Materials, 2021, 415: 125595 Chen Q L, Cui H L, Su J Q, et al. Antibiotic resistomes in plant microbiomes[J]. Trends in Plant Science, 2019, 24(6): 530-541 Zhang Z Y, Zhang Q, Wang T Z, et al. Assessment of global health risk of antibiotic resistance genes[J]. Nature Communications, 2022, 13(1): 1553 Rossi F, Rizzotti L, Felis G E, et al. Horizontal gene transfer among microorganisms in food: Current knowledge and future perspectives[J]. Food Microbiology, 2014, 42: 232-243 Buchholz U, Bernard H, Werber D, et al. German outbreak of Escherichia coli O104: H4 associated with sprouts[J]. New England Journal of Medicine, 2011, 365(19): 1763-1770 -
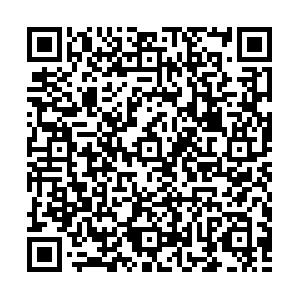
计量
- 文章访问数: 893
- HTML全文浏览数: 893
- PDF下载数: 185
- 施引文献: 0