天然有机质对TiO2纳米颗粒与PCB-77藻类毒性影响及致毒机理
Effect and Mechanism of Natural Organic Matter on Toxicity of TiO2 Nanoparticles and PCB-77 to Algae
-
摘要: 为探究天然有机质(natural organic matter,NOM)对纳米二氧化钛(TiO2)与3,3',4,4'-四氯联苯(3,3',4,4'-tetrachlorobiphenyl,PCB-77)藻类毒性影响及致毒机理,以小球藻为实验受试生物,测定了纳米TiO2与PCB-77单独及复合暴露在NOM存在与不存在条件下对小球藻生长、细胞形态、活性氧(reactive oxygen species,ROS)和丙二醛(malondialdehyde,MDA)含量及超氧化物歧化酶(superoxide dismutase,SOD)和过氧化氢酶(catalase,CAT)活性的影响。结果表明,所有暴露组均对小球藻的生长有一定的抑制作用。团聚沉降实验结果说明,纳米TiO2在水环境中易团聚,PCB-77和NOM通过影响纳米TiO2团聚进而影响小球藻的生长,这些团聚体附着在藻细胞表面,导致细胞间相互遮蔽,影响藻细胞光合作用和细胞呼吸,进而影响藻细胞生长,与实时定量PCR检测基因的结果一致。用透射电子显微镜(transmission electron microscope,TEM)观察发现,纳米TiO2单独暴露与PCB-77复合暴露在NOM存在与不存在条件下,藻细胞周围附着纳米TiO2,有一部分进入藻细胞内部,使细胞膜破损、质壁分离、细胞器结构不清晰以及出现电子致密体和空泡结构,小球藻细胞形态遭到损坏,这是直接接触造成的物理损伤。通过测定藻细胞内ROS和MDA含量可知,暴露在纳米TiO2中产生ROS含量最高,所受的氧化损伤最大,氧化胁迫是纳米TiO2致毒机理之一。因此,NOM对纳米TiO2与PCB-77藻类致毒机理的影响归纳为吸附团聚作用所造成的遮蔽效应、直接接触的物理损伤和产生大量ROS造成的氧化损伤。Abstract: In order to explore the effect of natural organic matter (NOM) on algae toxicity induced by nano-TiO2 and PCB-77 and its toxic mechanism, Chlorella vulgaris was used as the experimental organism. The effects of both presence and absence of NOM on the growth, cell morphology, contents of reactive oxygen species (ROS) and malondialdehyde (MDA), activities of superoxide dismutase (SOD) and catalase (CAT) of Chlorella vulgaris were determined. The results showed that the growth of Chlorella vulgaris was inhibited in all exposure groups. The agglomeration and sedimentation experiments showed that nano-TiO2 was easy to agglomerate in water environment. PCB-77 and NOM affected the growth of Chlorella vulgaris by affecting the agglomeration of nano-TiO2. These aggregates attachment to the surface of algae cells, resulting in mutual shielding between cells, which affecting the photosynthesis and cell respiration of algae cells, and eventually the growth of algae cells. This was therefore consistent with the results of real-time quantitative PCR. Transmission electron microscope observation showed that when nano-TiO2 and PCB-77 were exposed alone or in combination with NOM, nano-TiO2 adhered around the algal cells, some of which entered into the algal cells, causing cell membrane damage and plasmolysis, unclear organelle structure, electron dense body and vacuole structure, and thus damaged morphology of Chlorella vulgaris cells. All of which were the physical damages caused by direct contact. By measuring the content of ROS and MDA in algae cells, it can be seen that the exposure of the nano-TiO2 lead to the highest content of ROS and the severest damage of the cells. Oxidative stress is one of the toxic mechanisms of nano-TiO2. Therefore, the toxic mechanism of nano-TiO2 and PCB-77 on algae with NOM can be summarized as follows:shielding effect caused by adsorption agglomeration, physical damage caused by direct contact and oxidation damage caused by excessive amount of ROS.
-
Key words:
- nano-TiO2 /
- natural organic matter /
- PCB-77 /
- Chlorella /
- toxic effects /
- toxic mechanism
-
-
Zheng D, Wang N, Wang X M, et al. Effects of the interaction of TiO2 nanoparticles with bisphenol A on their physicochemical properties and in vitro toxicity[J]. Journal of Hazardous Materials, 2012, 199-200:426-432 Yan J, Lin B C, Hu C L, et al. The combined toxicological effects of titanium dioxide nanoparticles and bisphenol A on zebrafish embryos[J]. Nanoscale Research Letters, 2014, 9(1):406 Serdar B, LeBlanc W G, Norris J M, et al. Potential effects of polychlorinated biphenyls (PCBs) and selected organochlorine pesticides (OCPs) on immune cells and blood biochemistry measures:A cross-sectional assessment of the NHANES 2003-2004 data[J]. Environmental Health, 2014, 13:114 Dong H, Su C Y, Xia X M, et al. Polychlorinated biphenyl quinone-induced genotoxicity, oxidative DNA damage and γ-H2AX formation in HepG2 cells[J]. Chemico-Biological Interactions, 2014, 212:47-55 Attia S M, Ahmad S F, Okash R M, et al. Aroclor 1254-induced genotoxicity in male gonads through oxidatively damaged DNA and inhibition of DNA repair gene expression[J]. Mutagenesis, 2014, 29(5):379-384 Liu H M, Amy G. Modeling partitioning and transport interactions between natural organic matter and polynuclear aromatic hydrocarbons in groundwater[J]. Environmental Science & Technology, 1993, 27(8):1553-1562 Ottofuelling S, Kammer F V D, Hofmann T. Nanoparticles in the aquatic environment-aggregation behavior of TiO2nanoparticles studied in a simplified aqueous test matrix (SAM)[J]. Journal of Geophysical Research, 2007, 9:08876 Sadiq I M, Pakrashi S, Chandrasekaran N, et al. Studies on toxicity of aluminum oxide (Al2O3) nanoparticles to microalgae species:Scenedesmus sp. and Chlorella sp.[J]. Journal of Nanoparticle Research, 2011, 13(8):3287-3299 Lin D H, Ji J, Long Z F, et al. The influence of dissolved and surface-bound humic acid on the toxicity of TiO2 nanoparticles to Chlorella sp.[J]. Water Research, 2012, 46(14):4477-4487 Blum D J W, Speece R E. Determining chemical toxicity to aquatic species[J]. Environmental Science & Technology, 1990, 24(3):284-293 Li X, Zhang T, Min X M, et al. Toxicity of aromatic compounds to Tetrahymena estimated by microcalorimetry and QSAR[J]. Aquatic Toxicology, 2010, 98(4):322-327 刘畅, 吴文娟, 李建宏, 等. 不同光强对阿特拉津和百草枯藻类毒性的影响[J]. 环境科学学报, 2014, 34(5):1339-1343 Liu C, Wu W J, Li J H, et al. Effects of different light intensities on the toxicities of atrazine and paraquat to algae[J]. Acta Scientiae Circumstantiae, 2014, 34(5):1339-1343(in Chinese)
Wang Z, Wang S, Peijnenburg W J G M. Prediction of joint algal toxicity of nano-CeO2/nano-TiO2 and florfenicol:Independent action surpasses concentration addition[J]. Chemosphere, 2016, 156:8-13 Long Z F, Ji J, Yang K, et al. Systematic and quantitative investigation of the mechanism of carbon nanotubes toxicity toward algae[J]. Environmental Science & Technology, 2012, 46(15):8458-8466 Zhang L Q, Lei C, Chen J J, et al. Effect of natural and synthetic surface coatings on the toxicity of multiwalled carbon nanotubes toward green algae[J]. Carbon, 2015, 83:198-207 Perreault F, Oukarroum A, Melegari S P, et al. Polymer coating of copper oxide nanoparticles increases nanoparticles uptake and toxicity in the green alga Chlamydomonas reinhardtii[J]. Chemosphere, 2012, 87(11):1388-1394 Cui Y, Liu W, Xie W P, et al. Investigation of the effects of perfluorooctanoic acid (PFOA) and perfluorooctane sulfonate (PFOS) on apoptosis and cell cycle in a zebrafish (Danio rerio) liver cell line[J]. International Journal of Environmental Research and Public Health, 2015, 12(12):15673-15682 Fan J H, Cui Y B, Zhou Y, et al. The effect of nutrition pattern alteration on Chlorella pyrenoidosa growth, lipid biosynthesis-related gene transcription[J]. Bioresource Technology, 2014, 164:214-220 Sun X, Xu N J, Jiang L Z, et al. Gene expression profiles of the heterotrophic microalga Chlorella pyrenoidosa F-9[J]. Genetics and Molecular Research, 2014, 13(4):8411-8420 Zhang S, Deng R, Lin D H, et al. Distinct toxic interactions of TiO2 nanoparticles with four coexisting organochlorine contaminants on algae[J]. Nanotoxicology, 2017, 11(9-10):1115-1126 Mishra S, Srivastava S, Tripathi R D, et al. Phytochelatin synthesis and response of antioxidants during cadmium stress in Bacopa monnieri L.[J]. Plant Physiology and Biochemistry, 2006, 44(1):25-37 Phyu Y L, Palmer C G, Warne M S J, et al. Assessing the chronic toxicity of atrazine, permethrin, and chlorothalonil to the cladoceran Ceriodaphnia cf. dubia in laboratory and natural river water[J]. Archives of Environmental Contamination and Toxicology, 2013, 64(3):419-426 Kabra A N, Ji M K, Choi J, et al. Toxicity of atrazine and its bioaccumulation and biodegradation in a green microalga, Chlamydomonas mexicana[J]. Environmental Science and Pollution Research, 2014, 21(21):12270-12278 Romih T, Jemec A, Novak S, et al. FTIR microscopy reveals distinct biomolecular profile of crustacean digestive glands upon subtoxic exposure to ZnO nanoparticles[J]. Nanotoxicology, 2016, 10(4):462-470 Yu C X, Irudayaraj J. Spectroscopic characterization of microorganisms by Fourier transform infrared microspectroscopy[J]. Biopolymers, 2005, 77(6):368-377 Lu W D, Alam M A, Pan Y, et al. A new approach of microalgal biomass pretreatment using deep eutectic solvents for enhanced lipid recovery for biodiesel production[J]. Bioresource Technology, 2016, 218:123-128 Gelfand P, Smith R J, Stavitski E, et al. Characterization of protein structural changes in living cells using time-lapsed FTIR imaging[J]. Analytical Chemistry, 2015, 87(12):6025-6031 da Silva Ferreira V, ConzFerreira M E, Lima L M T R, et al. Green production of microalgae-based silver chloride nanoparticles with antimicrobial activity against pathogenic bacteria[J]. Enzyme and Microbial Technology, 2017, 97:114-121 Zhao J, Wang Z Y, Dai Y H, et al. Mitigation of CuO nanoparticle-induced bacterial membrane damage by dissolved organic matter[J]. Water Research, 2013, 47(12):4169-4178 Zhu X D, Wang Y J, Qin W X, et al. Distribution of free radicals and intermediates during the photodegradation of polychlorinated biphenyls strongly affected by cosolvents and TiO2 catalyst[J]. Chemosphere, 2016, 144:628-634 Schmitt D, Kumke M, Seibel F, et al. The influence of natural organic matter (NOM) on the desorption kinetics of pyrene and naphthalene from quartz[J]. Chemosphere, 1999, 38(12):2807-2824 Aruoja V, Dubourguier H C, Kasemets K, et al. Toxicity of nanoparticles of CuO, ZnO and TiO2 to microalgae Pseudokirchneriella subcapitata[J]. Science of the Total Environment, 2009, 407(4):1461-1468 冀静. 腐殖酸对纳米颗粒藻类毒性的影响及机理[D]. 杭州:浙江大学, 2011:32-34 Ji J. The effect and its mechanism of humic acid on the algal toxicity of nanoparticles[D]. Hangzhou:Zhejiang University, 2011:32 -34(in Chinese)
-
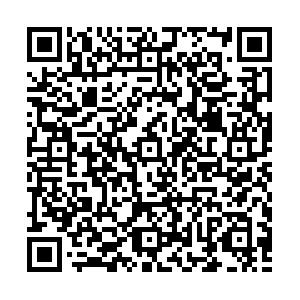
计量
- 文章访问数: 2168
- HTML全文浏览数: 2168
- PDF下载数: 102
- 施引文献: 0